The world of machining and engineering is filled with a multitude of technical terms and concepts that can often leave even the most experienced professionals scratching their heads. One such term is the “helix angle,” a crucial parameter in the design and performance of cutting tools and machinery. In this comprehensive guide, we will delve deep into the world of helix angles, explaining what they are, how to calculate them, and how they differ from lead angles.
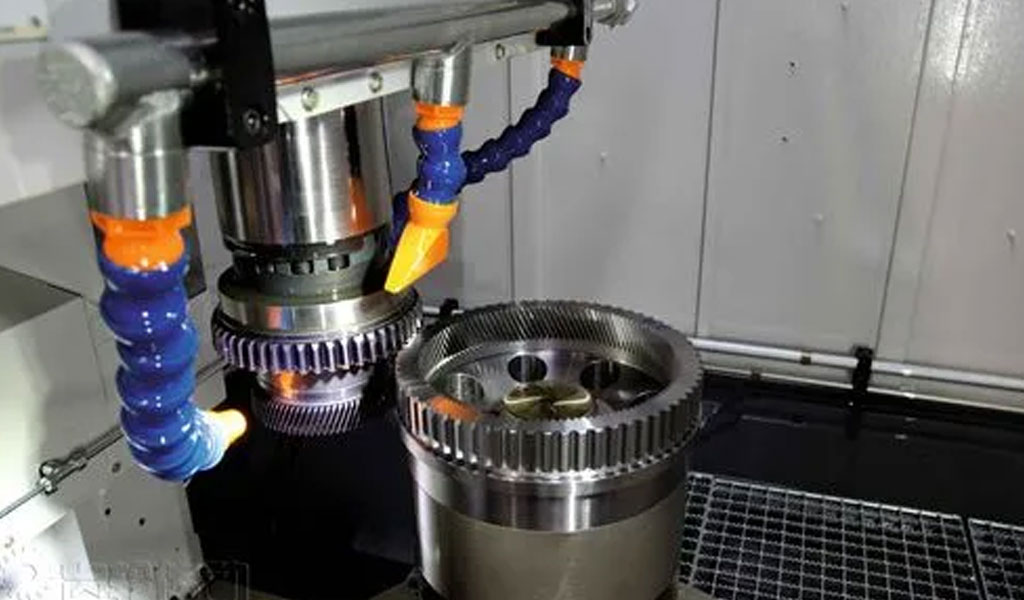
What Is Helix Angle
The helix angle, in the context of machining and engineering, refers to the angle formed between the helical cutting edge of a tool and a plane perpendicular to the axis of rotation or movement. It’s a fundamental geometric parameter that plays a pivotal role in determining how efficiently a cutting tool removes material during cnc machining operations.In simpler terms, imagine a drill bit or an end mill with flutes spiraling around its shaft. The angle at which these flutes or cutting edges spiral around the tool’s axis is the helix angle.
This angle is measured in degrees and is essential for understanding the tool’s behavior and performance in various cutting processes.
Importance of Helix Angle in Machining
The helix angle is of paramount importance in machining for several reasons:
- a. Chip Evacuation: One of its primary functions is to aid in chip evacuation. As a tool rotates and removes material, chips are generated. The helix angle helps guide these chips out of the cutting zone, preventing chip buildup that can lead to tool wear and poor surface finishes.
- b. Tool Strength: The helix angle also affects the tool’s strength and rigidity. A larger helix angle typically results in a more robust tool design, suitable for heavy-duty machining operations, while smaller angles might be preferred for lighter cuts and finer finishes.
- c. Tool Life: It significantly influences tool life. The right helix angle choice can reduce heat generation and distribute cutting forces evenly, prolonging the tool’s lifespan.
- d. Surface Finish: Helix angles impact the quality of the machined surface. In many cases, a specific helix angle can help reduce vibrations and chatter, resulting in a smoother finish.
Significance of Helix Angle in Various Applications
The importance of helix angles extends across various industries and machining applications:
- a. Metalworking: In metalworking, especially in milling and drilling operations, helix angles are crucial. They determine the tool’s performance in cutting materials like steel, aluminum, and titanium. Different materials may require different helix angles for optimal results.
- b. Woodworking: Woodworkers use tools with helix angles to create clean cuts and precise joinery. The angle can be adjusted to suit the wood’s hardness and grain.
- c. Aerospace: Helix angles are vital in aerospace cnc machining and other manufacturing, where precision and reliability are paramount. They impact the production of aircraft components, from engine parts to airframe components.
- d. Medical Device Manufacturing: In the medical device industry, where precision and surface finish are critical, helix angles play a role in producing intricate components like orthopedic implants and surgical instruments.
Historical Perspective on Helix Angles
The concept of helix angles has a rich historical background dating back to ancient times. Early civilizations, such as the Egyptians and Greeks, used simple tools with helical shapes for drilling and cutting tasks. However, it wasn’t until the Industrial Revolution that helix angles were rigorously studied and applied in machining processes.
In the 19th century, advancements in mathematics and engineering led to a deeper understanding of helical geometries. Engineers and scientists like Archimedes and Leonardo da Vinci laid the foundation for modern cutting tool design by exploring the principles of helical motion.
With the rise of manufacturing and the need for more efficient machining processes, helix angles became a focal point of research and development. Today, computer-aided design and simulation tools enable engineers to optimize helix angles for specific materials and applications, pushing the boundaries of what is achievable in machining technology. The historical journey of helix angles exemplifies how ancient principles continue to shape and enhance modern manufacturing practices.
What Is Lead Angle
The lead angle is a critical parameter in machining and manufacturing, closely related to the concept of helix angles. While both terms involve angles, they have distinct meanings and applications.
In simple terms, the lead angle refers to the angle formed between the direction of the tool’s motion or feed and a line perpendicular to the workpiece’s axis. This angle is typically measured in degrees and plays a crucial role in determining the efficiency and accuracy of machining operations.
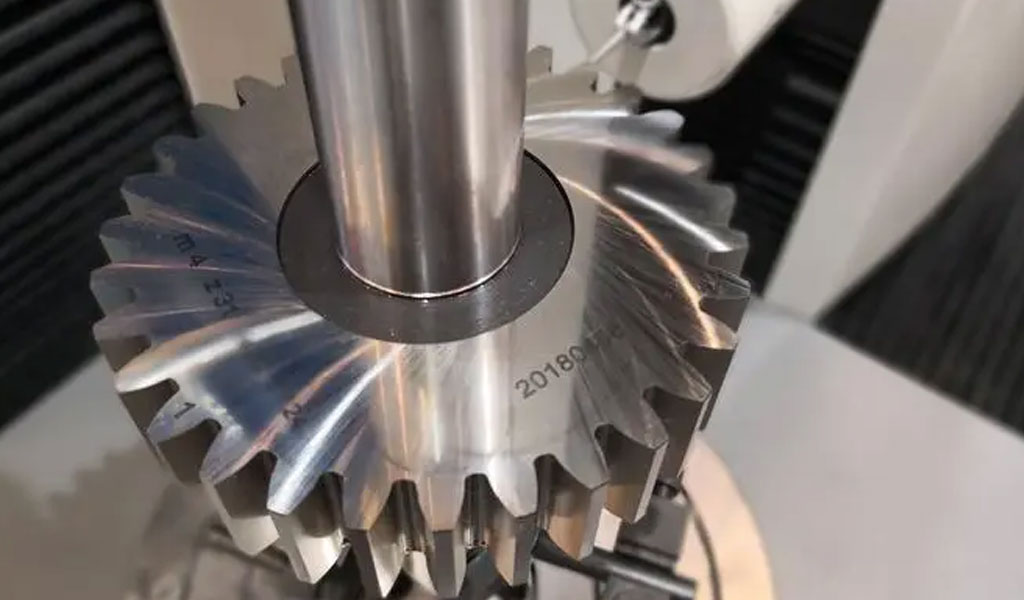
To illustrate the lead angle, consider a lathe, which is a common machining tool. As the cutting tool moves along the workpiece’s surface, the angle between the tool’s motion and a line perpendicular to the workpiece axis is the lead angle. It essentially describes how the tool advances or “leads” as it cuts into the material.
Lead Angle vs. Helix Angle: Clarifying the Differences
While both lead and helix angles involve angles and are relevant in machining, it’s important to understand their distinctions:
- a. Different Reference Lines: The key difference lies in the reference lines used to measure these angles. The helix angle is measured relative to the axis of rotation or movement of the cutting tool, while the lead angle is measured relative to the direction of tool motion in relation to the workpiece.
- b. Purpose and Impact: Helix angles primarily influence chip evacuation, tool strength, and surface finish. They determine how the cutting edges are oriented along the tool’s shaft and affect chip flow. On the other hand, lead angles are crucial for controlling the tool’s path and ensuring proper engagement with the workpiece. They influence the accuracy of machining operations, particularly in precision turning and threading processes.
- c. Machining Operation: Helix angles are more relevant in milling, drilling, and other operations where the tool rotates or spirals during cutting. Lead angles are predominantly important in turning, where the tool moves linearly along the workpiece’s surface.
Practical Applications of Lead Angles
The lead angle has several practical applications in machining and manufacturing:
- a. Turning Operations: In turning processes, the lead angle is essential for controlling the tool’s engagement with the workpiece. It helps determine the shape of the machined surface and influences factors like surface finish and tool wear. Adjusting the lead angle can optimize cutting conditions for different materials and workpiece geometries.
- b. Thread Cutting: Lead angles play a critical role in thread cutting operations. For instance, when creating external threads on a lathe, the lead angle corresponds to the angle of the thread helix. It ensures that the tool follows the correct path to create threads with the desired pitch and profile.
- c. Taper Turning: Taper turning involves machining a workpiece to create a conical or tapered shape. Lead angles are crucial in taper turning as they determine the rate at which the tool advances along the workpiece’s length and diameter. Accurate lead angle control is essential for achieving precise taper angles.
- d. Tool Selection: Lead angles are considered when selecting cutting tools. Different cutting tool geometries, such as those used in turning inserts, are designed with specific lead angles to suit various machining tasks. Choosing the right tool with the appropriate lead angle ensures efficient and accurate machining.
How Lead Angle and Helix Angle Interact in Machining Processes
Lead angles and helix angles often work together in certain machining processes, especially when turning with helical tool paths. Here’s how they interact:
- a. Combined Tool Geometries: Some cutting tools, particularly in turning, incorporate both lead and helix angles in their geometries. These tools are designed for operations where the tool moves linearly along the workpiece (lead angle) while simultaneously following a helical path (helix angle). This combination is useful for creating complex profiles or threads.
- b. Thread Milling: In thread milling, a helical tool path is used to create internal or external threads. The lead angle determines the rate of linear advancement along the workpiece, while the helix angle controls the spiral path of the tool. By synchronizing these angles, precise threads can be produced efficiently.
- c. Spiral Flute End Mills: In milling applications, end mills with spiral or helical flutes are commonly used. These end mills incorporate helix angles to aid in chip evacuation and surface finish. The lead angle, on the other hand, determines how the tool advances through the material. Properly selecting and coordinating these angles is essential for achieving the desired milling results.
While lead and helix angles are distinct concepts, they are closely intertwined in many machining processes. Understanding how these angles interact and how they impact the tool’s behavior is essential for achieving optimal machining results, whether you’re turning, milling, or threading materials in various manufacturing applications.
Calculating Helix Angle
The formula Helix angle = Atan (Lead of Screw/Circumference of Screw) or α= atan(L/C) is used to calculate the Helix Angle, which is represented by α symbol.
The Geometry of Helix Angle
Before we delve into the mathematical aspects of calculating helix angles, it’s essential to understand the geometry behind this critical machining parameter.
Imagine a cutting tool with flutes spiraling around its axis. The helix angle is the angle formed between the axis of the tool and a line perpendicular to the axis. To visualize this, picture a right triangle with the following elements:
- The hypotenuse represents the helical path traced by the cutting edge as the tool rotates.
- The adjacent side corresponds to the axial direction of the tool, running parallel to the tool’s axis.
- The opposite side represents the lateral movement or advancement of the tool as it progresses along the helical path.
The helix angle, denoted by the Greek letter “α” (alpha), is the angle formed between the adjacent side and the hypotenuse. This angle governs how the tool engages with the workpiece during cutting.
Mathematical Formulas for Helix Angle Calculation
To calculate the helix angle, you can use trigonometric principles, specifically the tangent function, as follows:
Tan(α) = (Opposite Side) / (Adjacent Side)
In this formula:
- “α” represents the helix angle (in radians).
- “Opposite Side” corresponds to the lateral movement of the tool, typically measured as the axial advancement per revolution.
- “Adjacent Side” is the axial distance the tool travels in one full rotation.
To convert the result from radians to degrees, you can use the following formula:
α (degrees) = α (radians) × (180° / π)
Now, let’s break down the process of calculating the helix angle step by step:
Step-by-Step Calculation Examples
Step 1: Measure the Axial Advancement (Opposite Side)
Begin by measuring the axial distance the tool travels in one full rotation. This distance represents the opposite side of the right triangle.
Step 2: Measure the Axial Distance (Adjacent Side)
Next, determine the axial distance or the length of the side of the right triangle that runs parallel to the tool’s axis. This value represents the adjacent side.
Step 3: Apply the Tangent Formula
Using the tangent formula mentioned earlier:
Tan(α) = (Opposite Side) / (Adjacent Side)
Plug in the values you measured in steps 1 and 2. Calculate the tangent of the helix angle.
Step 4: Calculate the Helix Angle in Radians (α radians)
To find the helix angle in radians, take the arctangent (inverse tangent) of the result from step 3:
α (radians) = arctan(Tan(α))
Step 5: Convert Radians to Degrees
If you prefer the helix angle in degrees, you can use the conversion factor:
α (degrees) = α (radians) × (180° / π)
Now that you understand the mathematical process for calculating the helix angle, let’s consider a practical example:
Example: Calculating the Helix Angle
Suppose you have a cutting tool that advances axially by 0.5 inches for every full revolution. The axial distance, representing the adjacent side, is 0.5 inches.
The lateral movement, representing the opposite side, is 0.2 inches.
Step 1: Measure the Axial Advancement (Opposite Side)
Opposite Side = 0.2 inches
Step 2: Measure the Axial Distance (Adjacent Side)
Adjacent Side = 0.5 inches
Step 3: Apply the Tangent Formula
Tan(α) = (0.2 inches) / (0.5 inches)
Tan(α) ≈ 0.4
Step 4: Calculate the Helix Angle in Radians (α radians)
α (radians) = arctan(0.4)
α (radians) ≈ 21.8 degrees
Step 5: Convert Radians to Degrees
α (degrees) = 21.8 degrees
So, the helix angle for this cutting tool is approximately 21.8 degrees.
Using Specialized Tools and Software for Helix Angle Calculation
While manual calculations as described above are informative, they may not be the most practical approach, especially in complex machining operations. In modern manufacturing, specialized tools and software can simplify and enhance the calculation of helix angles.
- Digital Calipers and Measuring Instruments: Precision measuring instruments like digital calipers and micrometers can provide accurate measurements of axial advancement and axial distance. These measurements can then be used in the trigonometric calculations.
- CAD/CAM Software: Computer-Aided Design (CAD) and Computer-Aided Manufacturing (CAM) software packages often include features for defining tool geometries, including helix angles. Engineers and machinists can input the tool parameters, and the software will calculate the helix angle automatically.
- Tool Manufacturers’ Documentation: Many cutting tool manufacturers provide comprehensive documentation for their products, including helix angles. This information can be found in catalogs, product specifications, or online resources. It eliminates the need for manual calculations.
- Online Calculators and Apps: Numerous online calculators and mobile apps are designed specifically for calculating helix angles and other machining parameters. These tools are user-friendly and efficient, making it easy to obtain accurate results.
While manual calculations using trigonometric principles are valuable for understanding the fundamentals of helix angles, modern machining practices often rely on specialized tools and software to streamline the process and ensure precision. Whether you prefer manual calculations or digital tools, having a grasp of helix angle calculation is essential for optimizing machining operations and achieving the desired outcomes in manufacturing.
Factors Influencing Helix Angle
Material Properties and Their Impact
The choice of material in machining processes has a significant influence on the selection and optimization of helix angles. Different materials exhibit varying mechanical properties, cutting behaviors, and responses to tool geometries. Here’s how material properties impact helix angle decisions:
- a. Hardness: The hardness of the material being machined affects the helix angle choice. Harder materials, like high-strength steels or ceramics, often require tools with larger helix angles. This is because a larger helix angle can reduce cutting forces, heat generation, and tool wear when machining hard materials.
- b. Ductility: Ductile materials, such as certain metals, tend to deform rather than fracture during machining. For ductile materials, a smaller helix angle may be preferred to maintain better chip control and surface finish. This reduces the risk of producing long, stringy chips that can wrap around the tool.
- c. Abrasiveness: Materials with abrasive properties, like composites or some ceramics, can accelerate tool wear. In such cases, optimizing the helix angle becomes crucial to distribute wear evenly along the cutting edge and extend tool life.
- d. Thermal Conductivity: Materials vary in their thermal conductivity. High thermal conductivity materials, like aluminum, dissipate heat more efficiently. Consequently, tool design, including helix angles, should consider heat generation and dissipation.
- e. Workpiece Size: The size and geometry of the workpiece can influence the choice of helix angle. For large workpieces, optimizing chip evacuation becomes more critical, potentially leading to different helix angle selections.
Tool Design and Helix Angle
Tool design plays a pivotal role in determining the appropriate helix angle for machining. The following factors highlight how tool design interacts with helix angle selection:
- a. Tool Material: The material from which the cutting tool is made can impact its ability to withstand the forces generated during machining. Tool materials vary in their toughness and wear resistance, influencing helix angle choices. For instance, carbide tools may allow for more aggressive helix angles due to their excellent wear resistance.
- b. Tool Geometry: Tool geometry, including the number of flutes and their design, affects chip evacuation and surface finish. Helix angles must complement the overall tool geometry to ensure efficient material removal and avoid chip-related issues.
- c. Coatings: Many cutting tools are coated with specialized coatings like titanium nitride (TiN) or diamond-like carbon (DLC) to enhance performance. The presence of coatings can impact friction and heat generation, which, in turn, may influence helix angle selection.
- d. Tool Rigidity: The rigidity of the cutting tool is essential for maintaining dimensional accuracy and surface finish. Tool design should align with the selected helix angle to ensure stability during machining, especially in high-speed or high-feed operations.
- e. Tool Wear and Chip Control: The helix angle can affect tool wear and chip control. Tools with optimized helix angles can reduce heat generation and chip entanglement, extending tool life and improving machining efficiency.
Machining Processes and Helix Angle
Different machining processes have distinct requirements and considerations when it comes to helix angle selection. Here’s how various processes interact with helix angles:
- a. Milling: In milling operations, especially in end milling or face milling, the helix angle impacts chip evacuation and surface finish. The choice of helix angle can help prevent chip recutting, reduce tool wear, and achieve smoother finishes.
- b. Drilling: Drilling operations, such as deep hole drilling, benefit from helix angles designed for efficient chip removal. A well-chosen helix angle ensures that chips are effectively cleared from the hole, preventing chip packing and drill breakage.
- c. Turning: In turning, the helix angle influences the tool’s engagement with the workpiece. A properly selected helix angle can improve chip control and surface finish. It’s especially crucial in applications like taper turning, where precise control of the tool’s path is necessary.
- d. Threading: Thread cutting processes require careful consideration of the helix angle to achieve accurate thread profiles. The helix angle corresponds to the thread’s pitch, and a mismatch can lead to threading errors.
- e. Reaming: Reaming operations involve enlarging existing holes to precise dimensions. The helix angle of the reamer affects the cutting action and surface finish of the hole. Proper helix angle selection ensures dimensional accuracy.
- f. Boring: Boring involves enlarging and finishing holes with a single-point tool. Helix angles play a role in controlling chip formation and optimizing surface finish in boring operations.
The Role of Cutting Speed, Feed Rate, and Depth of Cut
The cutting parameters, including cutting speed, feed rate, and depth of cut, interact closely with helix angle selection to influence machining performance:
- a. Cutting Speed: The cutting speed determines the rate at which the tool contacts the workpiece. It affects heat generation and tool wear. Adjusting the helix angle can help optimize chip formation and heat dissipation at different cutting speeds.
- b. Feed Rate: The feed rate controls the rate at which the tool advances into the workpiece. Balancing the helix angle with the feed rate is crucial for achieving efficient material removal without overloading the tool or workpiece.
- c. Depth of Cut: The depth of cut determines how much material is removed in each pass. It influences the chip size, cutting forces, and tool engagement. Proper helix angle selection ensures effective chip evacuation and minimizes tool wear at various depths of cut.
- d. Material Removal Rate (MRR): The combination of cutting speed, feed rate, depth of cut, and helix angle influences the material removal rate. Optimizing these parameters ensures efficient machining processes and maximizes productivity.
- e. Tool Life: The interplay of cutting parameters and helix angle affects tool life. A well-balanced approach can extend tool life by reducing wear, minimizing heat generation, and preventing chip-related issues.
- f. Surface Finish: Helix angle choices impact surface finish quality. By aligning the helix angle with the cutting parameters, you can achieve the desired surface finish while maintaining productivity.
Helix angle selection in machining is not an isolated decision but rather an integral part of a complex system involving material properties, tool design, machining processes, and cutting parameters. Engineers and machinists must carefully consider these factors to optimize tool performance, tool life, surface finish, and overall machining efficiency. The synergy between these elements ensures that helix angles are chosen to meet the specific requirements of each machining operation and material being processed.
Helix Angle in Practical Applications
Machining in the Aerospace Industry
The aerospace industry is characterized by the need for precision, reliability, and strict adherence to quality standards. Helix angles play a critical role in ensuring that components used in aircraft, spacecraft, and related equipment meet these stringent requirements. Here’s a closer look at the application of helix angles in aerospace machining:
- a. Turbine Blade Manufacturing: Turbine blades used in jet engines demand intricate profiles and tight tolerances. The helix angles of cutting tools are carefully selected to optimize material removal while minimizing heat generation. The proper choice of helix angle ensures the performance and longevity of these critical components.
- b. Aircraft Structural Components: Components such as wing spars and fuselage parts are often made from high-strength materials like aluminum and titanium. Helix angles are essential for milling and drilling operations on these components. The correct helix angle selection helps maintain component integrity, reduces machining costs, and ensures strict dimensional tolerances.
- c. Composite Materials: Modern aircraft increasingly incorporate composite materials for their lightweight and high-strength properties. These materials, including carbon-fiber-reinforced composites, pose unique machining challenges. Helix angles are crucial in minimizing delamination, fraying, and surface defects when machining composites.
- d. Engine Component Fabrication: Helix angles are especially critical in manufacturing engine components such as shafts, casings, and bearing housings. The right helix angle selection ensures efficient material removal, precision machining, and reduced cycle times. It also minimizes the risk of workpiece and tool damage.
- e. Heat-Resistant Alloys: Aerospace materials often include heat-resistant alloys like Inconel and superalloys. These alloys are notoriously difficult to machine due to their high hardness and tendency to work harden. Helix angles must be carefully chosen to manage cutting forces, heat, and tool wear during machining.
- f. Precision Machining for Instrumentation: Instruments and avionics systems used in aerospace require high-precision components. Helix angles play a crucial role in producing intricate parts with tight tolerances, ensuring the accuracy and reliability of these instruments.
In the aerospace industry, where safety and performance are paramount, the application of helix angles is a key factor in achieving the required quality and efficiency in machining processes. Aerospace manufacturers invest in advanced tooling and machining strategies that incorporate optimal helix angles to meet stringent industry standards.
Automotive Manufacturing and Helix Angles
The automotive manufacturing sector relies heavily on precision machining to produce complex components for vehicles. Helix angles are integral to achieving the desired quality and efficiency in various aspects of automotive production:
- a. Engine Block Machining: The engine block is a critical component in an automobile’s powertrain. Machining processes, including boring and milling, require well-selected helix angles to optimize material removal and surface finish. Proper helix angle choices contribute to engine block reliability and performance.
- b. Gear Manufacturing: Helical gears are commonly used in automotive transmissions. The helix angle of the gear-cutting tools determines the gear’s tooth profile and performance characteristics. Accurate helix angles are essential for smooth gear engagement and transmission efficiency.
- c. Suspension Components: Suspension components, such as control arms and struts, require precise machining for optimal performance and safety. The helix angle affects the tool’s path and material removal rate during turning and milling operations on these parts.
- d. Brake System Components: Brake rotors and calipers undergo machining processes to meet dimensional tolerances and surface finish requirements. Helix angles influence chip control, tool wear, and surface finish during these machining operations.
- e. Chassis Fabrication: The chassis is the structural backbone of a vehicle. Helix angles play a role in machining chassis components like frame rails and crossmembers. Proper helix angle selection ensures that these components meet strength and dimensional specifications.
- f. Engine Component Manufacturing: Automotive engines comprise various components, from crankshafts to camshafts. Helix angles are carefully chosen to optimize the machining of these critical parts, ensuring their longevity and performance.
- g. Precision Machining for Electric Vehicles (EVs): The growing popularity of electric vehicles requires precision machining of components like battery casings and electric motors. Helix angles contribute to the efficient machining of these specialized parts.
In the automotive industry, where production volumes are high, cost efficiency and quality control are paramount. Helix angles are part of the equation for achieving efficient machining processes that meet strict standards for performance, safety, and reliability.
Medical Device Fabrication: A Case Study
Medical device fabrication demands exceptional precision and attention to detail. Helix angles play a crucial role in machining intricate components used in medical instruments and implants. Let’s explore a case study to understand the significance of helix angles in this industry:
Case Study: Manufacturing Orthopedic Implants
Orthopedic implants, such as hip and knee prostheses, require precision machining to meet the specific needs of patients. Helix angles are critical in the production of these implants:
- a. Material Selection: Medical implants are typically made from biocompatible materials like titanium or cobalt-chromium alloys. The hardness and characteristics of these materials necessitate tooling with specific helix angles to minimize heat generation and ensure precise machining.
- b. Complex Geometries: Implants often feature intricate geometries, such as threads or custom contours. The helix angle of the cutting tools influences the accuracy of machining these complex features.
- c. Surface Finish: Implants must have smooth and defect-free surfaces to minimize the risk of infection and ensure patient comfort. Helix angles are carefully chosen to achieve the desired surface finish without compromising dimensional accuracy.
- d. Batch Production: Medical device manufacturers often produce implants in batches. Optimizing helix angles ensures consistent and efficient machining processes, reducing production time and costs.
- e. Tool Wear Management: Extended tool life is crucial in medical device machining to maintain consistent quality and minimize production interruptions. Proper helix angle selection helps manage tool wear and reduces the frequency of tool changes.
In the medical device fabrication industry, precision and biocompatibility are non-negotiable. Helix angles are part of the comprehensive approach to machining orthopedic implants and other medical components that meet exacting standards for quality, safety, and patient well-being.
Helix Angles in Woodworking and Carpentry
Helix angles are not limited to metalworking; they also find application in woodworking and carpentry. The choice of helix angles is vital in shaping and finishing wood products. Here’s how helix angles come into play in this industry:
- a. Router Bits and Woodworking Tools: Woodworkers use various tools, including router bits and spiral-cutting tools, to shape wood. The helix angle of these tools affects chip evacuation and the quality of the cut. By selecting the appropriate helix angle, woodworkers can achieve clean, splinter-free cuts and intricate profiles.
- b. Drilling and Boring: Helix angles are significant in drilling and boring holes in wood. They influence the efficiency of chip removal, preventing clogs and ensuring accurate hole dimensions. Helix angles also play a role in counterboring and countersinking operations.
- c. Woodturning: Woodturning involves rotating a piece of wood while cutting tools shape it into various forms, such as bowls and spindles. The helix angle of lathe tools affects the tool’s approach to the workpiece and the quality of the turned surface.
- d. CNC Woodworking: Computer Numerical Control (CNC) machining has become prevalent in woodworking. CNC routers equipped with tools featuring specific helix angles enable precise and efficient machining of wood panels and components used in furniture and cabinetry.
- e. Chip Control: Efficient chip control is crucial in woodworking to prevent workpiece damage, tool wear, and poor surface finish. The choice of helix angle helps ensure that chips are evacuated smoothly, contributing to the overall quality of the woodworking project.
In woodworking and carpentry, where aesthetics and precision are essential, the careful selection of helix angles is fundamental to achieving the desired results. Woodworkers and craftsmen rely on their knowledge of helix angles to create intricate designs and high-quality wood products.
Advanced Topics in Helix Angle
Variable Helix Angles: Purpose and Benefits
Variable helix angles represent an advanced concept in machining, offering flexibility and precision in various cutting applications. Unlike constant helix angles, which maintain a consistent angle along the cutting tool’s length, variable helix angles change gradually or in a controlled manner. This dynamic approach to helix angles introduces unique advantages:
Purpose of Variable Helix Angles:
The primary purpose of variable helix angles is to optimize cutting performance across different regions of a tool, a workpiece, or machining conditions. By adjusting the helix angle along the tool’s length, engineers and machinists can address specific challenges and requirements in a machining process.
Benefits of Variable Helix Angles:
- Reduced Vibration and Chatter: One of the significant benefits is the reduction of vibration and chatter during machining. Variable helix angles help distribute cutting forces more evenly, enhancing tool stability and surface finish.
- Improved Tool Life: By tailoring the helix angle to different machining conditions, tool wear can be minimized. This leads to extended tool life and reduced tool replacement costs.
- Enhanced Chip Evacuation: Variable helix angles facilitate efficient chip evacuation. In areas with difficult chip control, a steeper helix angle can aid in chip removal, preventing chip buildup and potential tool damage.
- Surface Finish Optimization: Variable helix angles allow for the optimization of surface finish in specific regions of a workpiece. Finer finishes can be achieved where needed, enhancing part quality.
- Adaptation to Material Variations: When machining materials with varying properties, such as composite materials or castings, variable helix angles enable adjustments to accommodate the changing material characteristics.
- Minimized Heat Generation: In some applications, reducing heat generation is crucial to prevent workpiece material distortion or premature tool wear. Variable helix angles can help achieve this by controlling the cutting forces and heat dissipation.
- Versatility: Variable helix angles make tools more versatile, suitable for a broader range of materials and applications. This versatility reduces the need for frequent tool changes.
Examples of Variable Helix Angle Tools:
- Twisted End Mills: These end mills feature a helical twist along their flutes, resulting in a variable helix angle. Twisted end mills are commonly used for milling applications and excel at reducing chatter and improving surface finish.
- Variable Helix Drills: Drills with variable helix angles are effective in reducing drilling vibrations and enhancing hole quality. They are suitable for various materials, including metals and composites.
- Variable Pitch Reamers: Reamers with variable helix angles and pitch adjustments are used to fine-tune bore dimensions and achieve precise tolerances. They are valuable in applications requiring tight fits.
- Variable Helix Taps: Variable helix taps are designed to minimize tapping-related issues like chip clogging and oversize or undersize threads. They improve thread quality and extend tap life.
Variable helix angle tools are particularly valuable in high-performance machining, where precision, tool life, and surface finish are critical. By customizing the helix angle to match specific machining conditions and workpiece materials, manufacturers can achieve superior results and operational efficiency.
Multiflute Tools and Helix Angle Variations
Multiflute tools are an integral part of modern machining, offering increased productivity and enhanced performance. They often incorporate variations in helix angles, providing several advantages in cutting processes. Here’s an in-depth look at multiflute tools and their helix angle variations:
Multiflute Tool Basics:
Multiflute tools are cutting tools, such as end mills and drills, that have multiple cutting edges or flutes. They are designed for various machining operations, including milling, drilling, and reaming. Multiflute tools have gained popularity due to their ability to remove material more efficiently and maintain better tool stability.
Helix Angle Variations in Multiflute Tools:
Variations in helix angles within multiflute tools can offer several benefits:
- Improved Chip Evacuation: Different helix angles along the flutes of a multiflute tool can help break up and evacuate chips more effectively. This is particularly advantageous in deep-hole drilling and high-speed milling, where chip control is essential to prevent tool wear and workpiece damage.
- Enhanced Tool Stability: Variations in helix angles can help distribute cutting forces more evenly across the cutting edges. This improved stability reduces the risk of vibration, chatter, and tool deflection, leading to better part quality and longer tool life.
- Surface Finish Optimization: By adjusting the helix angle in specific sections of the tool, manufacturers can optimize the surface finish in critical areas of the workpiece. This is valuable in applications where surface quality is a priority.
- Reduction in Heat Generation: Variations in helix angles can be used strategically to control heat generation during machining. In areas where excessive heat can lead to workpiece distortion or tool wear, a shallower helix angle can be employed to minimize heat buildup.
Examples of Multiflute Tools with Helix Angle Variations:
- Variable Helix End Mills: These end mills feature flutes with varying helix angles. The combination of shallow and steep helix angles optimizes chip removal, minimizes chatter, and improves surface finish. Variable helix end mills are commonly used in milling applications.
- Step Drills: Step drills often incorporate variations in helix angles to efficiently drill different-sized holes in a single operation. The varying angles help control chip evacuation and maintain dimensional accuracy.
- Specialized Reamers: Reamers designed for precision hole finishing may feature variations in helix angles to enhance bore quality. These reamers are essential for achieving tight tolerances in critical applications.
- Customized Cutting Tools: Some manufacturers offer custom multiflute tools with specific helix angle variations to address unique machining challenges. These tools are tailored to meet the exact requirements of the application.
Multiflute tools with helix angle variations are versatile and adaptable to a wide range of machining scenarios. They are particularly valuable in industries that demand high precision, efficient material removal, and extended tool life.
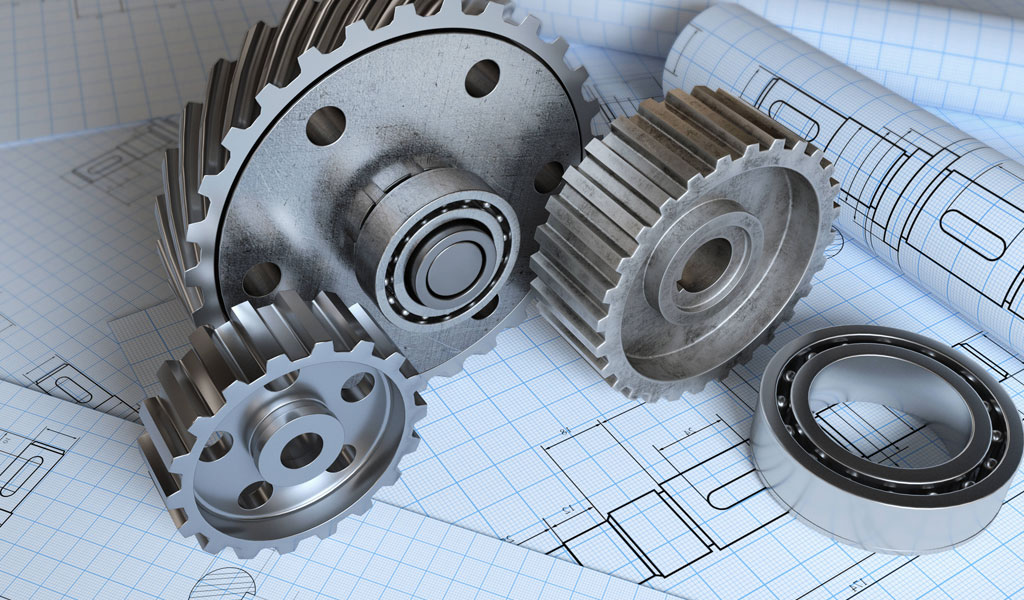
Helical Milling: Techniques and Strategies
Helical milling is an advanced machining technique that utilizes the helix angle of cutting tools to optimize material removal and improve machining efficiency. This method is especially valuable in high-speed machining and the production of complex parts. Let’s explore the techniques and strategies associated with helical milling:Helical milling involves rotating a cutting tool while simultaneously advancing it along a helical path. The helix angle of the tool determines the direction and pitch of this spiral path. During helical milling, chips are evacuated more efficiently than in traditional milling, leading to several benefits:
Benefits of Helical Milling:
- Improved Chip Evacuation: The helical path of the tool helps guide chips away from the cutting zone more effectively. This reduces the risk of chip recutting, chip entanglement, and heat buildup.
- Enhanced Surface Finish: Helical milling can result in a smoother surface finish due to reduced tool-to-workpiece contact and improved chip control. This is particularly valuable in applications where surface quality is critical.
- Reduced Cutting Forces: The gradual engagement of the tool in helical milling reduces cutting forces, leading to less tool wear, lower power consumption, and increased tool life.
- Enhanced Tool Rigidity: Helical milling can enhance tool rigidity, especially in long-reach applications, by distributing cutting forces more evenly along the length of the tool.
Helical Milling Techniques and Strategies:
- Ramping In and Out: Helical milling often involves ramping into and out of a workpiece. This gradual approach reduces the shock to the tool and workpiece and minimizes the risk of tool breakage.
- Contouring and Pocketing: Helical milling is commonly used for contouring and pocketing operations. The tool follows a helical path while machining complex shapes and cavities, ensuring efficient chip removal.
- Variable Helix Angles: Some helical milling tools have variable helix angles along their length. These variations optimize performance in different regions of the toolpath, addressing specific machining challenges.
- High-Speed Machining (HSM): Helical milling is well-suited for high-speed machining, where the combination of reduced cutting forces and improved chip evacuation leads to enhanced productivity and part quality.
- Coolant and Lubrication: Proper coolant and lubrication are crucial in helical milling to manage heat and prevent workpiece and tool damage. Coolant delivery should be directed effectively to reach the cutting zone.
- Tool Selection: Selecting the right cutting tool with the appropriate helix angle and geometry is critical for successful helical milling. Tool manufacturers offer a range of helical milling tools designed for various applications.
- CNC Programming: CNC programs for helical milling must specify the helix angle, pitch, and toolpath strategy. Accurate programming ensures that the tool follows the intended helical path.
Helical milling is a versatile technique that finds applications in aerospace, automotive, mold and die making, and other industries that demand efficient material removal and precise part geometry. When properly executed, helical milling can result in significant improvements in machining performance and overall productivity.
Tool Wear and Maintenance Considerations
In advanced machining processes, such as those involving variable helix angles, multiflute tools, and helical milling, tool wear and maintenance become crucial aspects of efficient and cost-effective manufacturing. Here are key considerations for managing tool wear and maintenance in advanced machining:
Tool Wear Mechanisms:
- Flank Wear: This is the most common type of tool wear and occurs on the tool’s flank or side surface due to friction between the tool and the workpiece material.
- Crater Wear: Crater wear appears on the tool’s rake face and results from high-temperature interactions between the tool and the workpiece material.
- Adhesive Wear: Adhesive wear occurs when workpiece material adheres to the tool’s cutting edge, leading to material transfer and tool deterioration.
- Abrasive Wear: Abrasive wear is caused by the presence of hard particles in the workpiece material, which gradually erode the tool’s cutting edges.
Strategies for Managing Tool Wear:
- Proper Tool Selection: Choosing the right tool material and coating is critical. Advanced coatings, such as TiAlN (Titanium Aluminum Nitride) or TiCN (Titanium Carbonitride), can significantly enhance tool life and performance.
- Optimized Cutting Speeds and Feeds: Adjusting cutting speeds and feed rates to match the workpiece material and machining conditions helps minimize tool wear. High-speed machining techniques can also reduce wear rates.
- Coolant and Lubrication: Effective coolant and lubrication systems are essential for dissipating heat and preventing excessive tool wear. Tailor coolant delivery to reach the cutting zone and control temperature.
- Tool Path Optimization: Employing advanced toolpath strategies, such as trochoidal milling or adaptive machining, can reduce tool wear by distributing cutting forces more evenly and avoiding abrupt changes in direction.
- Monitoring and Inspection: Regularly inspecting tools for signs of wear and using tool monitoring systems can help detect wear-related issues early, allowing for timely tool changes and maintenance.
Tool Maintenance Considerations:
- Tool Reconditioning: Depending on the type of wear and the tool’s condition, some tools can be reconditioned through processes like regrinding or recoating, extending their service life.
- Tool Replacement: In cases of severe wear or tool damage, timely tool replacement is necessary to maintain machining precision and productivity.
- Storage and Handling: Proper tool storage in designated containers or racks and careful handling to prevent nicks or damage are essential for tool longevity.
- Inventory Management: Implementing an effective tool inventory management system ensures that replacement tools are readily available when needed, reducing downtime.
- Tool Life Analysis: Conducting tool life analysis and recording data, such as tool wear rates, helps in developing predictive maintenance strategies and optimizing tool usage.
- Operator Training: Training operators in tool maintenance and handling procedures is vital to prevent mishandling or misuse that can accelerate tool wear.
Advanced machining processes often involve high-performance tools and materials, making tool wear and maintenance management critical to achieving consistent results and minimizing production costs. By implementing effective strategies and staying proactive in tool maintenance, manufacturers can extend tool life, reduce downtime, and enhance overall machining efficiency.
Cutting-Edge Technologies and Helix Angles
Computer-Aided Design (CAD) and Helix Angles
Computer-Aided Design (CAD) is a cornerstone of modern engineering and manufacturing processes. It plays a pivotal role in optimizing helix angles by enabling engineers to visualize, design, and simulate components and toolpaths. Here’s how CAD contributes to helix angle optimization:
- Visualization and Design:Geometry Modeling: CAD software allows engineers to create 3D models of workpieces, cutting tools, and machine setups. This modeling capability provides a detailed view of components and their interactions, including helix angles;Tool Selection: Engineers can choose cutting tools with specific helix angles from a library of tool designs available in CAD software. This selection aligns with the requirements of the machining task and workpiece material.
- Simulation and Analysis:Toolpath Simulation: CAD software can simulate toolpath movements, taking into account the helix angle. Engineers can visualize how the tool engages with the workpiece, helping identify potential issues such as collisions or inefficient cutting paths;Material Removal Simulation: By simulating material removal processes, engineers can analyze chip formation, tool wear, and surface finish based on the chosen helix angle. This analysis informs decisions about optimal helix angles for specific applications.
- Iterative Design and Optimization:Parametric Modeling: CAD software often supports parametric modeling, enabling engineers to make changes to the design and immediately see the effects on helix angles and other parameters. This iterative process helps refine designs for better machining outcomes;Design Validation: Engineers can use CAD simulations to validate the chosen helix angles before physical machining begins. This reduces the risk of errors and costly rework in the manufacturing process.
- Integration with CAM:Computer-Aided Manufacturing (CAM) software often works in tandem with CAD systems. Engineers can export CAD models to CAM software, which generates toolpaths based on helix angles, cutting conditions, and workpiece geometry. This seamless integration streamlines the transition from design to machining.
Numerical Control (CNC) Machining and Helix Angle Optimization
Numerical Control (CNC) machining has revolutionized the precision and flexibility of machining operations. It plays a vital role in helix angle optimization by enabling real-time control and adjustment of cutting parameters. Here’s how CNC machining contributes to helix angle optimization:
- Dynamic Control:Toolpath Execution: CNC machines precisely follow programmed toolpaths, including those involving helix angles. This dynamic control ensures that the tool maintains the intended helix angle throughout the machining process;Feed Rate Adjustment: CNC controllers can adjust feed rates based on real-time feedback from sensors and tool wear monitoring systems. This capability helps maintain optimal helix angles by adapting to changing cutting conditions.
- Multi-Axis Machining:5-Axis Machining: CNC machines with 5-axis capabilities provide enhanced freedom to control the tool’s orientation and helix angle during machining. This is particularly advantageous in complex and contoured surfaces;Simultaneous 5-Axis Machining: Simultaneous 5-axis machining enables continuous tool movement along multiple axes, allowing for intricate helix angle adjustments in real-time. It is crucial for complex parts with varying geometries.
- Toolpath Optimization:Adaptive Toolpaths: Advanced CNC software offers adaptive toolpath strategies that optimize helix angles based on the tool’s engagement with the workpiece. These strategies reduce vibrations, improve surface finish, and extend tool life;Trochoidal Milling: Trochoidal milling techniques involve circular toolpaths with varying helix angles. CNC machines can execute these toolpaths efficiently, minimizing tool wear and heat generation.
- Integration with CAD/CAM:CNC machining is closely integrated with CAD/CAM systems. Engineers can create CNC programs that incorporate the desired helix angles and toolpath strategies defined during the design and simulation phases. This integration ensures consistency between design intent and machining execution.
- Real-Time Monitoring:Tool Wear Sensors: CNC machines equipped with tool wear sensors can monitor cutting tool conditions during machining. When tool wear exceeds predefined thresholds, the CNC controller can adjust feed rates or toolpath strategies to maintain optimal helix angles.
Artificial Intelligence and Machine Learning in Helix Angle Prediction
Artificial Intelligence (AI) and Machine Learning (ML) are transforming machining processes, including the prediction and optimization of helix angles. These technologies offer data-driven insights and real-time adaptability in helix angle selection. Here’s how AI and ML are advancing helix angle prediction:
- Data Analytics:Historical Data Analysis: AI and ML algorithms can analyze historical machining data, including tool wear, surface finish, and helix angle choices. This analysis identifies patterns and correlations to inform future helix angle selections;Material Property Assessment: AI can process data related to workpiece material properties, such as hardness and thermal conductivity, to suggest optimal helix angles for specific materials. This data-driven approach enhances machining efficiency and tool life.
- Real-Time Adaptation:Sensor Integration: CNC machines equipped with sensors for temperature, vibration, and cutting forces can provide real-time data. AI algorithms process this data to adjust helix angles and cutting parameters on the fly, optimizing machining efficiency and tool wear;Predictive Analytics: AI-driven predictive models can forecast the best helix angle choices based on current machining conditions, workpiece material, and tool wear status. This proactive approach minimizes errors and maximizes performance.
- Toolpath Optimization:Dynamic Toolpath Adjustment: AI can dynamically adjust toolpaths and helix angles based on real-time feedback. For example, if a sensor detects vibrations or excessive heat, AI algorithms can adapt the toolpath to maintain stability and tool integrity.
- Customized Helix Angle Recommendations:Machine Learning Models: ML models trained on vast datasets can provide customized helix angle recommendations for specific machining scenarios, workpiece materials, and tool types. These recommendations optimize machining outcomes.
- Reducing Trial and Error:Virtual Machining Simulations: AI-powered simulations enable engineers to explore different helix angle scenarios virtually. This reduces the need for costly trial-and-error experimentation on the shop floor.
- Tool Life Prediction:Predictive Maintenance: AI algorithms can predict tool wear and tool failure based on machining data and historical trends. By anticipating tool wear, CNC machines can automatically adjust helix angles or pause machining for tool replacement, minimizing downtime.
AI and ML technologies are revolutionizing the machining industry by providing intelligent, data-driven insights into helix angle selection and optimization. As these technologies continue to evolve, manufacturers can expect increased precision, efficiency, and overall productivity in their machining processes.
In Conclusion
In this comprehensive exploration of helix angles in machining, we’ve uncovered a wealth of knowledge and insights. Here’s a brief recap of the key takeaways from this article:
- Definition of Helix Angle: Helix angle is the angle formed between the cutting edge of a tool or drill bit and a plane perpendicular to the axis of rotation. It is a critical parameter in machining operations that significantly impacts chip formation, tool wear, surface finish, and overall machining efficiency.
- Importance of Helix Angle: Helix angles are essential for optimizing machining processes across various industries, including aerospace, automotive, medical device fabrication, and woodworking. They play a vital role in chip control, tool stability, surface quality, and tool life.
- Lead Angle vs. Helix Angle: Lead angle and helix angle are related but distinct concepts. Lead angle pertains to the advancement of a tool along its axis, affecting the pitch of threads in threading operations. Helix angle, on the other hand, focuses on the tool’s orientation during machining, influencing chip formation and tool engagement.
- Helix Angle Calculation: Calculating helix angles involves understanding the geometry of the tool, the number of flutes, and the lead of the tool. Mathematical formulas and step-by-step examples illustrate how to compute helix angles accurately.
- Factors Influencing Helix Angle: Material properties, tool design, machining processes, cutting parameters, and workpiece size all influence helix angle decisions. Tailoring the helix angle to these factors is crucial for achieving optimal machining results.
- Practical Applications: Helix angles find practical applications in diverse industries, including aerospace, automotive manufacturing, medical device fabrication, and woodworking. They contribute to the efficiency, precision, and quality of machining processes in each domain.
- Advanced Topics: Advanced concepts like variable helix angles, multiflute tools, helical milling, and AI-driven helix angle optimization are pushing the boundaries of machining capabilities. These innovations offer benefits such as reduced vibration, enhanced tool life, and real-time adaptive control.
- Computer-Aided Design (CAD) and CNC Machining: CAD software aids in the visualization, design, and optimization of helix angles. CNC machining systems seamlessly incorporate helix angle data into toolpath programming, enabling precise control and adaptability.
- Artificial Intelligence and Machine Learning: AI and ML technologies are revolutionizing helix angle prediction, optimization, and real-time control. These data-driven approaches enhance machining efficiency, tool life, and overall productivity.
The Ongoing Relevance of Helix Angles
As manufacturing technologies continue to evolve, the relevance of helix angles in machining remains undiminished. Helix angles are fundamental to achieving the delicate balance between efficiency, precision, and cost-effectiveness in various industries. With advancements in tool design, CNC machining, and AI-driven optimization, helix angles are becoming even more versatile and adaptable to the complex demands of modern manufacturing.
The aerospace and automotive sectors rely on helix angles to produce components that meet stringent standards for safety, performance, and reliability. In medical device fabrication, helix angles are instrumental in crafting intricate and biocompatible implants. Woodworkers and carpenters utilize helix angles to shape and finish wood products with precision.
Innovations such as variable helix angles, multiflute tools, and helical milling techniques are pushing the boundaries of what’s achievable in machining. These advancements offer manufacturers new ways to optimize tool performance, reduce downtime, and enhance product quality.
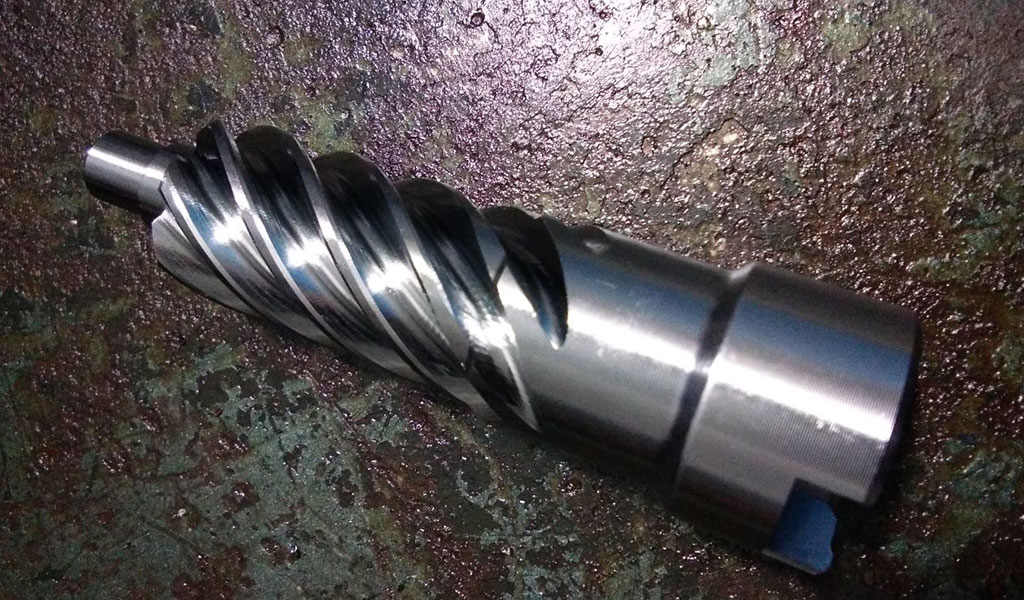
Artificial intelligence and machine learning are transforming helix angle prediction and optimization, providing valuable insights and real-time control. These technologies empower manufacturers to make data-driven decisions, adapt to changing conditions, and explore new possibilities in machining.
Encouragement for Further Exploration and Experimentation
As we conclude this article, we encourage engineers, machinists, and researchers to continue their exploration and experimentation with helix angles in machining. The field of machining is dynamic, with ongoing developments in tooling, technology, and methodologies. Here are some avenues for further exploration:
- Advanced Materials: With the emergence of new materials, such as advanced composites and high-strength alloys, the role of helix angles in optimizing machining processes will continue to evolve. Investigate how helix angles can be tailored to the properties of these materials.
- Customized Tooling: Explore the possibilities of customized tool designs that incorporate unique helix angle configurations to address specific machining challenges. Experiment with multiflute tools and variable helix angles to achieve superior results.
- Smart Machining Systems: Embrace the integration of AI and ML into machining processes. Develop and implement AI-driven solutions that optimize helix angles in real-time, adapting to changing conditions and materials.
- Sustainability: Consider the environmental impact of machining processes. Investigate how helix angles can be optimized to reduce energy consumption, waste generation, and tooling requirements.
- Interdisciplinary Collaboration: Collaborate across disciplines, such as materials science, mechanical engineering, and computer science, to unlock innovative approaches to helix angle optimization.
- Education and Training: Invest in education and training programs to empower the next generation of machinists and engineers with the knowledge and skills needed to harness the full potential of helix angles in machining.
In conclusion, helix angles are a cornerstone of modern manufacturing, enabling the creation of precise, efficient, and high-quality products across diverse industries. Their continued relevance and evolution hold the promise of further advancements in machining technology and the realization of new possibilities in the world of manufacturing. Embrace the challenges and opportunities that helix angles offer, and let innovation drive the future of machining.