Thread milling is a versatile and efficient machining process that has revolutionized the way threads are created in a wide range of industries. Instead of relying on traditional tapping methods, thread milling employs specialized tools and principles to cut precise threads in a variety of materials, from cnc machining metals to plastics. In this comprehensive article, we will delve into the world of thread milling, exploring its various types, the underlying principles, and the numerous advantages it offers.
View More Articles:
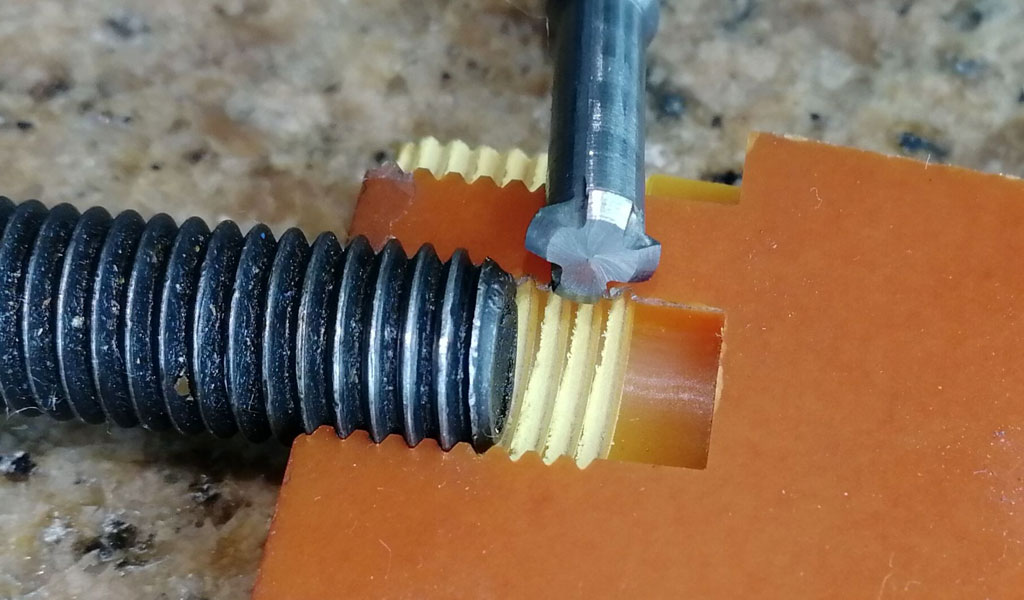
Thread Milling Basics
Thread milling is a machining technique that plays a pivotal role in modern engineering and manufacturing processes. It has become the preferred method for creating threads in a wide range of materials, offering several advantages over traditional threading methods.
In this section, we will delve into the basics of thread milling, starting with an understanding of threads and their significance in engineering and manufacturing.
A. Understanding Threads
Threads are helical ridges or grooves that spiral around the exterior or interior of cylindrical objects, typically referred to as the workpiece. They are designed to facilitate the joining or fastening of components, ensuring a secure and reliable connection. Threads serve a multitude of essential functions in engineering and manufacturing:
- Fastening and Assembly: Threads are used to join components together, such as nuts and bolts or screws and threaded holes. This allows for easy assembly, disassembly, and maintenance of various structures and machines.
- Load Distribution: Threads distribute mechanical loads evenly across the engaged components, enhancing the structural integrity of connections and preventing shear forces.
- Sealing and Leakage Prevention: In applications where fluids or gases are involved, threads can be designed to create a seal, preventing leaks and ensuring the containment of substances.
- Adjustability: Threads enable fine adjustments in mechanical systems, such as the focus on a camera lens or the tension of a guitar string.
- Measurement and Calibration: Threads are used in measurement instruments, like micrometers, to precisely gauge the dimensions of objects.
There are two primary categories of threads: internal threads and external threads.
- Internal Threads: These threads are found inside a hole or recess in a workpiece. Common examples include the threads inside a nut or the threads in a tapped hole.
- External Threads: These threads are located on the outside surface of a cylindrical workpiece. Examples include the threads on a screw or a bolt.
To standardize the design and manufacturing of threads, various thread profiles have been established. Two of the most widely used standards are the Unified Thread Standard (UTS) and the Metric Thread Standard.
- Unified Thread Standard (UTS): Also known as the Unified National Thread, this standard is commonly used in the United States and Canada. It features a combination of inch-based thread sizes and pitch values, making it versatile for a wide range of applications. The UTS includes various thread series, such as Unified National Thread, Unified Fine Thread, and Unified Extra Fine Thread.
- Metric Thread Standard: The Metric Thread Standard is prevalent in many parts of the world, especially in Europe and Asia. It utilizes the metric system, featuring thread sizes specified in millimeters. The most commonly used metric thread series is the ISO metric thread, which includes coarse and fine thread options.
B. Traditional Thread Production Methods
Before the widespread adoption of thread milling, traditional methods were employed to create threads, each with its limitations and challenges.
Tapping: Tapping is a conventional method for producing internal threads, typically in drilled holes. It involves using a tap, a cutting tool with threads matching the desired thread profile. The tap is rotated into the hole, cutting threads as it progresses. Tapping has several limitations, including:
Limited tool life: Taps wear out relatively quickly, especially when used in tough materials like stainless steel or hardened steel.
Difficulty in deep holes: Tapping long or deep holes can be challenging due to chip evacuation issues and increased torque requirements.
Limited versatility: Taps are specific to particular thread sizes and profiles, necessitating a separate tap for each application.
Single-Point Threading: Single-point threading is a manual or CNC machining process used for both internal and external threads. It involves feeding a single-point cutting tool, such as a lathe tool or threading tool, along the workpiece’s axis to create the thread. While this method offers more flexibility than tapping, it also has its challenges:
Slower production: Single-point threading can be time-consuming, especially for long threads or large quantities.
Setup complexity: Achieving precise thread profiles and tolerances often requires meticulous setup and tool positioning.
Limited to straight threads: Single-point threading is typically used for straight threads and may not be suitable for complex thread profiles.
C. Introduction to Thread Milling
Thread milling emerged as a revolutionary alternative to traditional thread production methods, offering greater precision, versatility, and efficiency. Let’s explore the key aspects of thread milling:
A Brief History of Thread Milling: Thread milling has been in use for several decades, but its popularity surged with the advent of CNC machining. The ability to program intricate thread paths and the development of specialized thread mill cutters significantly expanded its applications.
Key Components and Terminology:
- Thread Mill: The core tool used in thread milling, the thread mill is a cutting tool with multiple cutting edges. These cutting edges engage with the workpiece to create threads.
- Workpiece: The workpiece is the material in which threads are being milled. It can be a solid block, a pre-drilled hole, or a component in a larger assembly.
- Cutter Path: The cutter path refers to the tool’s movement as it traverses the workpiece to create threads. It can be a helical path, circular path, or a combination of both, depending on the desired thread profile.
The Role of CNC Machining in Modern Thread Milling: CNC (Computer Numerical Control) machining plays a crucial role in modern thread milling operations. CNC machines are capable of precisely controlling the movement of the thread mill cutter, ensuring accurate thread dimensions and profiles. CNC programming allows for the creation of complex and customized thread patterns, making thread milling suitable for a wide array of applications.
In the following sections of this article, we will delve deeper into the various types of thread mills, the principles governing thread milling, and the manifold advantages that this machining technique offers in the realm of engineering and manufacturing.
Types of Thread Mills
Thread mills come in various forms and designs, each tailored to specific applications and threading requirements. Understanding these different types is essential for selecting the right tool for the job. In this section, we’ll explore four main categories of thread mills: single-form thread mills, multi-form thread mills, solid carbide thread mills, and indexable thread mills.
A. Single-Form Thread Mills
Single-form thread mills are the most basic type of thread milling cutters. They are designed to create threads with a single profile, meaning they cut a consistent thread geometry throughout the entire milling process.
Description and Design Principles:
Single-form thread mills consist of a cylindrical body with helical flutes or cutting edges along its length. The flutes are shaped to match the specific thread profile desired. For example, if you need to mill a 60-degree V-shaped thread, the flutes will have a V-shaped cross-section. These cutters may have a single, double, or multiple flutes, depending on the thread size and application.
Applications and Advantages:
- General Threading: Single-form thread mills are suitable for a wide range of threading applications, including creating common thread profiles like ISO metric threads, Unified National Threads, and others.
- Simple Threads: They are ideal for straightforward thread profiles with no complex features or variations.
Single-Form Thread Milling Examples:
- ISO Metric Threads: Single-form thread mills are commonly used to produce metric threads, which follow the metric thread ISO standard. These threads are prevalent in many industries worldwide, particularly in Europe and Asia.
- Unified National Threads: In the United States and Canada, Unified National Threads are widely used, and single-form thread mills are employed to create these threads. These include Unified National Thread, Unified Fine Thread, and Unified Extra Fine Thread.
B. Multi-Form Thread Mills
Multi-form thread mills are designed to create threads with multiple profiles or features within a single milling pass. These cutters are highly versatile and can tackle complex threading requirements efficiently.
Design Variations and Customization Options:
Multi-form thread mills are characterized by their intricate flute designs. Instead of a uniform flute shape, they have flutes that change along their length to match the desired thread profile. This variation allows them to cut different portions of the thread simultaneously.
Use Cases in Complex Threading:
- Multi-Start Threads: Multi-form thread mills are perfect for machining multi-start threads, where multiple threads run parallel to each other on a single workpiece. Examples include ACME threads and worm gears.
- Special Thread Profiles: When dealing with non-standard or custom thread profiles, multi-form thread mills can create intricate designs with precision.
Advantages over Single-Form Thread Mills:
- Efficiency: Multi-form thread mills can complete complex threads in a single pass, reducing machining time significantly compared to single-form cutters, which require multiple passes to achieve similar results.
- Accuracy: These cutters maintain high accuracy and consistency, even when dealing with intricate thread profiles.
- Reduced Tool Changes: Since multi-form thread mills can handle a wider range of thread profiles, there’s less need for tool changes, streamlining the machining process.
C. Solid Carbide Thread Mills
Solid carbide thread mills are designed for durability and performance in challenging machining environments. They are constructed from solid carbide, a hard and wear-resistant material that can withstand the rigors of machining tough materials.
Material Composition and Properties:
Solid carbide thread mills are made from a composite material primarily consisting of tungsten carbide (WC) particles held together with a cobalt (Co) binder. This combination of materials provides several key properties:
- Hardness: Tungsten carbide is exceptionally hard, ensuring long tool life even when machining abrasive materials.
- Wear Resistance: Carbide tools are highly resistant to wear, making them suitable for high-speed machining and extended use.
- Heat Resistance: Solid carbide can withstand high temperatures generated during machining without losing its cutting edge hardness.
Benefits for Specific Applications:
- Hard Materials: Solid carbide thread mills are ideal for machining hard materials such as stainless steel, titanium, and hardened steel.
- High-Speed Machining: Their wear resistance and heat tolerance make them suitable for high-speed machining operations, improving productivity.
Maintenance and Tool Life Considerations:
While solid carbide thread mills offer many advantages, they require proper maintenance to maximize their tool life. Regular tool inspection, proper speeds and feeds, and coolant use are essential to preserve the longevity and performance of these cutters.
D. Indexable Thread Mills
Indexable thread mills are designed with replaceable cutting inserts, making them economical and practical choices for thread milling operations.
Introduction to Indexable Inserts:
Indexable thread mills consist of a cutter body that holds indexable inserts with cutting edges. These inserts are replaceable, allowing for cost-effective tool maintenance. When an insert becomes worn or damaged, it can be swapped out for a new one, extending the tool’s life.
Configurations and Threading Possibilities:
Indexable thread mills come in various configurations, including single-point and multi-point options. The choice of inserts and their positioning on the cutter body determines the threading possibilities.
Economical and Practical Advantages:
- Cost-Efficiency: The ability to replace inserts instead of the entire tool reduces tooling costs over time. This is particularly advantageous in high-volume production settings.
- Flexibility: Different inserts can be used to create various thread profiles with the same cutter, enhancing flexibility in manufacturing.
- Reduced Downtime: When an insert is replaced, there’s minimal downtime compared to regrinding and reconditioning solid carbide thread mills.
Thread milling offers a range of options to suit various threading needs, from single-form cutters for basic threads to multi-form cutters for complex profiles. Solid carbide thread mills excel in demanding applications, while indexable thread mills provide a cost-effective solution for high-volume production. Understanding the characteristics and advantages of each type allows manufacturers to make informed decisions when selecting the right tool for their specific threading requirements.
Thread Milling Principles
Thread milling is a precision machining process that relies on specific tool geometry, cutter paths, speeds and feeds, and programming to create accurate and high-quality threads. In this section, we will explore the fundamental principles that govern thread milling operations.
A. Tool Geometry
The quality of threads produced during thread milling largely depends on the tool geometry. Understanding the anatomy of a thread mill cutter and the parameters such as helix angle, pitch, and lead is essential for achieving precise thread profiles.
Anatomy of a Thread Mill Cutter:
A thread mill cutter consists of several key components:
- Shank: The shank is the non-cutting portion of the tool, designed for insertion into the tool holder of a milling machine.
- Flutes: Flutes are the cutting edges of the thread mill. They extend from the shank and are responsible for removing material to create threads.
- Helix Angle: The helix angle refers to the angle between the flutes and the centerline of the tool. It determines the shape of the threads being cut. Common helix angles include 30 degrees, 45 degrees, and 60 degrees, among others.
- Cutter Diameter: This is the diameter of the tool, which influences the size of the threads produced.
- Cutter Length: The cutter length determines how deep the threads can be milled in a single pass.
- Flute Shape: The shape of the flutes corresponds to the desired thread profile. For instance, V-shaped flutes are used for creating V-thread profiles.
Helix Angle, Pitch, and Lead:
- Helix Angle: The helix angle, as mentioned earlier, is the angle at which the flutes spiral around the cutter. It directly influences the shape of the threads. A larger helix angle results in a steeper thread, while a smaller angle produces a shallower thread.
- Pitch: Pitch is the distance between corresponding points on adjacent threads in a parallel thread configuration. It is usually expressed in millimeters (for metric threads) or threads per inch (for imperial threads). For example, a pitch of 1.0 mm means that the distance between threads is 1.0 mm.
- Lead: Lead, on the other hand, is the axial distance a screw thread advances in one complete rotation. It is the product of the pitch and the number of thread starts. For single-start threads, lead equals pitch.
Importance of Tool Geometry for Thread Quality:
Proper tool geometry is critical for achieving high-quality threads. The following aspects highlight its importance:
- Thread Profile Accuracy: The helix angle and flute shape must match the desired thread profile precisely. Any deviation can result in inaccuracies or thread imperfections.
- Thread Depth Control: Cutter length plays a vital role in controlling the depth of the threads. Incorrect cutter length can lead to under or over-milling, affecting thread quality.
- Chip Evacuation: Effective chip evacuation is essential to prevent chip buildup, which can lead to tool damage and poor thread quality. The tool’s flute design should facilitate efficient chip removal.
- Durability: Proper tool geometry also affects tool durability. An appropriately designed cutter is less likely to experience premature wear and breakage, ensuring consistent performance.
B. Cutter Paths and Toolpaths
Understanding the movement of the thread mill cutter and the various cutter paths and toolpaths available is crucial for achieving accurate threads with the desired profile.
Understanding Cutter Movement:
In thread milling, the cutter moves along a predefined path to remove material and create threads. Key considerations in cutter movement include:
- Axial Movement: This refers to the cutter’s movement along the axial (Z-axis) direction of the workpiece. It determines the thread depth.
- Radial Movement: Radial movement, along the radial (X and Y) directions, shapes the thread’s diameter and profile.
- Rotational Movement: The thread mill cutter rotates as it moves, which is essential for creating the helical shape of the threads.
Types of Cutter Paths:
- Helical Cutter Paths: Helical cutter paths are the most common for thread milling. The cutter follows a helical trajectory while simultaneously rotating to create the threads. This method is suitable for most thread profiles and offers excellent control over thread depth.
- Circular Cutter Paths: Circular cutter paths involve circular motions with the cutter. They are typically used for circular thread profiles or when specialized thread forms are required.
- Spiral Cutter Paths: Spiral cutter paths combine both radial and axial movements in a spiral pattern. They are used for generating threads with intricate geometries or non-standard thread profiles.
Optimization for Various Thread Profiles:
The choice of cutter path and toolpath depends on the specific thread profile being produced. Manufacturers must select the most suitable path and program it accurately to achieve the desired thread geometry. Additionally, toolpath optimization can minimize tool wear and enhance machining efficiency.
C. Speeds and Feeds
Properly calculating and implementing speeds and feeds in thread milling operations is essential for achieving optimal performance and extending tool longevity. In this section, we will delve into the details of how to calculate rotational speed (RPM) and feed rate (IPM), the factors that affect these parameters, and strategies for achieving the best results.
Calculating Rotational Speed (RPM) and Feed Rate (IPM):
Rotational Speed (RPM):The rotational speed of the thread mill cutter is a critical parameter that determines how fast the tool spins during the machining process. To calculate the RPM, you can use the following formula:RPM = (CS x 1,000) / (π x Cutter Diameter)Where:
RPM is the rotational speed in revolutions per minute.
CS (Cutting Speed) is the surface speed of the workpiece material, typically measured in meters per minute (m/min) or feet per minute (ft/min).
π (pi) is approximately 3.14159.
Cutter Diameter is the diameter of the thread mill cutter in the same units as CS.It’s important to note that the cutting speed (CS) is specific to the material being machined. Different materials require different cutting speeds for optimal performance. Cutting speed data can be found in machining reference tables or databases.
Feed Rate (IPM):The feed rate represents the linear speed at which the thread mill cutter moves along the workpiece. It is typically measured in inches per minute (IPM). The formula to calculate the feed rate is as follows:IPM = RPM x Pitch x Number of FlutesWhere:
IPM is the feed rate in inches per minute.
RPM is the rotational speed calculated using the previous formula.
Pitch is the thread pitch, usually in inches for imperial threads or millimeters for metric threads.
Number of Flutes corresponds to the number of cutting edges on the tool.Calculating the feed rate accurately is crucial to ensure that the tool advances at the proper rate during thread milling.
Factors Affecting Speeds and Feeds:
Several factors can affect the selection of cutting speeds and feed rates in thread milling operations:
- Material Type: Different materials have varying cutting speed requirements. Hard materials generally require lower cutting speeds, while softer materials can be machined at higher speeds.
- Tool Material: The type of material from which the thread mill cutter is made can affect the recommended speeds and feeds. Solid carbide cutters, for instance, can tolerate higher speeds compared to high-speed steel (HSS) cutters.
- Tool Diameter: The cutter diameter directly impacts the rotational speed (RPM) and feed rate (IPM) calculations. Larger diameter cutters typically require lower RPMs for the same cutting speed.
- Tool Geometry: The tool’s helix angle, flute design, and coatings can influence chip evacuation and, consequently, the feed rate. Properly designed tools can help achieve optimal chip formation and removal.
- Workpiece Material Hardness: Harder materials often require slower cutting speeds and may necessitate adjustments to prolong tool life.
Achieving Optimal Performance and Tool Longevity:
To ensure the best results in thread milling while maximizing tool longevity, consider the following strategies:
- Select the Right Tool Material: Choose a thread mill cutter made from the appropriate material for the workpiece material. Solid carbide cutters offer excellent wear resistance and are suitable for machining a wide range of materials.
- Tool Coatings: Some thread mill cutters come with specialized coatings that enhance performance. Coatings can reduce friction and heat generation, leading to longer tool life and improved machining efficiency.
- Tool Maintenance: Regularly inspect the tool for wear, damage, or dullness. Replace worn or damaged cutters promptly to maintain thread quality and extend tool life.
- Coolant Usage: Use coolant effectively to reduce heat and friction during machining. Proper coolant flow helps in chip evacuation and extends tool life.
- Cutting Parameters Optimization: Adjust cutting parameters, including speeds and feeds, to reduce cutting forces and prevent tool wear. Optimize these parameters based on the specific material and thread profile being machined.
- Testing and Monitoring: Conduct test runs and monitor the machining process closely, especially when working with new materials or thread profiles. Make adjustments as needed to achieve optimal results.
- Machine Rigidity: Ensure that the machining equipment is rigid and properly maintained. Machine stability and accuracy play a significant role in achieving consistent results.
D. Programming Thread Milling
Efficient programming is essential for successful thread milling operations. This section covers key aspects of programming thread milling, including G-code commands, toolpath generation using Computer-Aided Manufacturing (CAM) software, and the importance of simulation and verification.
G-code Commands for Thread Milling:
G-code is a language used to control CNC machines and specify tool movements. When programming thread milling, you’ll need to incorporate G-code commands that define the toolpath and machining parameters. Here are some essential G-code commands commonly used in thread milling:
- Tool Selection: Use G-code commands to select the appropriate tool from the tool library.
- Tool Change: Include commands to initiate tool changes when necessary.
- Tool Compensation: Implement tool compensation commands to account for tool diameter and length offsets, ensuring accurate thread geometry.
- Feedrate Control: Set the feedrate (IPM) using G-code commands to control how fast the tool moves along the workpiece.
- Rotational Speed: Specify the rotational speed (RPM) of the spindle using G-code commands to achieve the desired cutting speed.
- G-code for Helical Movements: Define helical movements, including the pitch and number of turns, to create the thread profile. Helical moves are a fundamental component of thread milling.
- Coolant Control: Use G-code commands to control the coolant system, ensuring proper cooling and chip evacuation during machining.
Toolpath Generation and CAM Software:
While you can manually program thread milling using G-code, it’s often more efficient and error-resistant to use Computer-Aided Manufacturing (CAM) software. CAM software streamlines the programming process by providing a user-friendly interface for defining toolpaths, specifying cutting parameters, and simulating the machining process.
Here’s an overview of the steps involved in programming thread milling with CAM software:
- Import CAD Geometry: Start by importing the CAD model of the workpiece into the CAM software. This allows you to visualize the machining process and generate toolpaths based on the geometry.
- Toolpath Generation: Define the thread milling operation by selecting the appropriate tool, specifying cutting parameters (speeds and feeds), and generating toolpaths. CAM software often offers predefined thread milling strategies for various thread profiles.
- Simulation: Before sending the program to the CNC machine, simulate the machining process within the CAM software. Simulation allows you to identify potential issues, verify tool clearances, and ensure that the toolpaths are correctly generated.
- Post-Processing: After successful simulation, the CAM software generates the G-code program specific to your thread milling operation. This G-code can then be loaded onto the CNC machine’s control unit.
Simulation and Verification:
Simulation is a critical step in programming thread milling operations. It helps identify any programming errors, collisions, or issues that could lead to tool breakage or damage to the workpiece. During simulation, pay attention to the following aspects:
- Verify that the toolpaths are correctly aligned with the workpiece geometry.
- Check for any tool collisions with clamps, fixtures, or other machine components.
- Ensure that the tool does not exceed its designed limits in terms of depth and width of cut.
- Confirm that the feedrates and rotational speeds are within the specified parameters.
Additionally, it’s advisable to perform a dry run (without the actual workpiece) on the CNC machine to verify the program’s accuracy before machining the final workpiece. This step allows for real-time monitoring and adjustment of the machining process.
Proper calculation and implementation of speeds and feeds are crucial for successful thread milling operations. By accurately calculating rotational speed (RPM) and feed rate (IPM) while considering factors like material type, tool geometry, and machine rigidity, you can optimize tool performance and achieve high-quality threads. Additionally, utilizing CAM software for programming, simulation, and verification streamlines the process and reduces the likelihood of errors, contributing to efficient and accurate thread milling operations.
Advantages of Thread Milling
Thread milling has become a preferred machining method for creating threads in a wide range of industries. Its numerous advantages over traditional threading methods, such as tapping and single-point threading, have made it a go-to choice for precision, versatility, and cost-efficiency. In this section, we’ll explore the key advantages of thread milling in detail.
A. Precision and Accuracy
One of the primary advantages of thread milling is its ability to consistently produce threads with exceptional precision and accuracy. This precision is crucial in various industries and applications where tight tolerances are a requirement.
Achieving Tight Tolerances:
- Consistent Thread Pitch: Thread milling ensures that the thread pitch remains uniform throughout the entire threaded region. This consistency is essential for components like screws, where the pitch must meet exact specifications.
- Repeatability: CNC-controlled thread milling machines allow for high repeatability, ensuring that each threaded component matches the desired specifications with minimal variation.
Consistency in Thread Quality:
- Reduced Variability: Thread milling minimizes the risk of variations and defects that can occur with traditional threading methods. This consistency is crucial in applications where thread performance is critical, such as in automotive and aerospace components.
- Accurate Thread Profiles: Thread mills are designed with specific tool geometries to match the desired thread profile precisely. This accuracy results in threads that meet the intended design standards.
Reducing Scrap and Rework:
- Less Material Waste: The precision of thread milling means there is less material waste compared to traditional methods that may require multiple passes to achieve accurate threads.
- Minimized Rework: Accurate threads from the outset mean fewer components that require rework or correction, leading to cost savings and increased efficiency in manufacturing.
B. Versatility in Materials
Thread milling offers the flexibility to work with a wide range of materials, including various metals, plastics, and composites. This versatility is particularly advantageous in industries where different materials are used for specific applications.
Thread Milling in Various Materials:
- Metals: Thread milling can be applied to a wide variety of metals, including steel, stainless steel, aluminum, brass, and titanium. This adaptability makes it suitable for industries such as automotive, aerospace, and general manufacturing, where different metals are used for various components.
- Plastics: Thread milling is equally effective when working with plastics. It provides a clean, precise method for creating threads in materials like nylon, polyethylene, and polycarbonate, commonly used in industries like consumer electronics and medical devices.
- Composites: Thread milling can handle composite materials, which are increasingly used in aerospace and other advanced applications. The ability to work with composites like carbon fiber-reinforced polymers (CFRPs) allows for lightweight, strong threaded components.
High-Performance Materials and Exotic Alloys:
- Stainless Steel and Hardened Alloys: Thread milling can effectively machine tough materials like stainless steel and hardened alloys. This capability is invaluable in industries such as oil and gas, where corrosion-resistant materials are essential.
- Exotic Alloys: In aerospace and defense applications, thread milling can handle exotic alloys like Inconel and titanium, known for their high strength and heat resistance.
Minimizing Tool Wear:
Thread milling cutters, especially those made of solid carbide, exhibit excellent wear resistance. This characteristic allows them to maintain consistent cutting performance even when working with abrasive or hard materials. As a result, tool life is extended, reducing the need for frequent tool changes and associated downtime.
C. Cost-Efficiency
While precision and material versatility are vital aspects of thread milling, cost-efficiency is another significant advantage that makes it an attractive choice for manufacturers.
Comparing Costs with Traditional Threading Methods:
- Reduced Material Costs: Thread milling minimizes material waste since it can create precise threads in a single pass. In contrast, traditional methods may require multiple passes, leading to greater material wastage.
- Decreased Labor Costs: Thread milling is highly automated when performed with CNC machines, reducing labor requirements compared to manual threading methods.
- Tooling Costs: While thread milling cutters may have higher upfront costs, their longevity and the reduced need for frequent tool changes often make them more cost-effective in the long run.
Tool Life and Maintenance Considerations:
- Extended Tool Life: High-quality thread milling cutters, especially those made from solid carbide, can withstand the rigors of machining abrasive materials, leading to extended tool life. This longevity reduces tool replacement costs and the associated downtime.
- Reduced Machine Wear: Thread milling generates less wear and tear on machines compared to traditional threading methods, leading to lower maintenance costs and increased machine longevity.
Long-Term Savings and ROI:
- Improved Productivity: Thread milling’s efficiency and precision result in faster production times and reduced scrap rates, leading to increased overall productivity.
- Higher Quality Products: The accuracy and consistency of thread milling contribute to the production of higher-quality products with fewer defects, reducing warranty claims and associated costs.
- Competitive Advantage: Companies that adopt thread milling as part of their manufacturing processes can gain a competitive edge by offering superior products with shorter lead times and lower production costs.
D. Complex Threads and Special Profiles
Thread milling excels in creating complex threads and special profiles that may be challenging or impossible to achieve using traditional threading methods. This advantage is particularly valuable in industries where specialized components with intricate thread designs are required.
Navigating Intricate Threading Requirements:
- Multi-Start Threads: Thread milling is ideal for creating multi-start threads, where multiple threads run parallel to each other on a single workpiece. Applications include ACME threads and worm gears, commonly used in machinery and power transmission systems.
- Special Thread Profiles: Thread milling can produce threads with non-standard profiles, such as trapezoidal threads or custom-designed threads tailored to specific applications. These custom profiles are invaluable in industries where standard threads do not suffice.
Custom and Non-Standard Thread Profiles:
- Aerospace Industry: In aerospace applications, where safety and precision are paramount, thread milling is often used to create unique and complex threads for components like aircraft engines and critical structural elements.
- Automotive Industry: Thread milling is employed in the automotive sector to produce specialized threads used in various components, including engine parts, transmissions, and suspension systems.
The advantages of thread milling, including precision, material versatility, cost-efficiency, and the ability to create complex threads, have positioned it as a key machining method in various industries. As technology continues to advance, thread milling is likely to play an even more significant role in the production of high-quality, precision components. Manufacturers that embrace thread milling stand to benefit from improved product quality, reduced production costs, and a competitive edge in the market.
Troubleshooting and Tips
Thread milling, while offering numerous advantages, is not without its challenges. In this section, we’ll explore common thread milling problems and provide best practices and tips for mitigating these issues.
A. Common Thread Milling Problems
Chip Evacuation Issues:Problem: Inadequate chip evacuation can lead to chip buildup around the cutter, causing tool damage and affecting thread quality.
Causes:
- Insufficient coolant flow.
- Incorrect cutting parameters (speeds and feeds).
- Poor chip design or tool geometry.
Solution:
- Ensure proper coolant flow and adequate chip clearance.
- Adjust cutting parameters for optimal chip formation and removal.
- Choose thread mills with effective chip evacuation features.
Tool Breakage and Wear:Problem: Tool breakage and wear can result from machining tough materials, improper tool selection, or inadequate maintenance.
Causes:
- Using the wrong tool for the material or application.
- Excessive cutting forces due to incorrect speeds and feeds.
- Neglecting tool maintenance.
Solution:
- Select the appropriate tool material and geometry for the material being machined.
- Optimize speeds and feeds to reduce cutting forces.
- Regularly inspect and maintain thread mill cutters, replacing them when necessary.
Thread Distortion and Size Variation:Problem: Thread distortion or size variation can occur when the thread mill cutter encounters excessive resistance or material inconsistencies.
Causes:
- Inadequate support or clamping of the workpiece.
- Material hardness variations.
- Excessive cutting forces.
Solution:
- Ensure proper workpiece support and secure clamping.
- Select appropriate cutting parameters to reduce forces.
- Verify material quality and consistency before machining.
B. Best Practices and Tips
Tool Selection and Maintenance:
- Choose the Right Cutter: Select the appropriate thread mill cutter based on material type, hardness, and thread profile requirements. Solid carbide cutters are suitable for demanding applications, while indexable cutters offer cost-efficiency.
- Tool Inspection: Regularly inspect the tool for wear, damage, or dullness. Replace worn or damaged cutters promptly to maintain thread quality and extend tool life.
- Coolant Usage: Use coolant effectively to reduce heat and friction during machining. Proper coolant flow helps in chip evacuation and extends tool life.
Programming and Setup:
- Accurate Programming: Ensure that the CNC program accurately defines the thread profile, cutter path, and cutting parameters. Thoroughly test and simulate the program before actual machining.
- Toolpath Optimization: Optimize toolpaths for efficient chip evacuation and reduced tool wear. Consider helical, circular, or spiral paths based on the thread profile and workpiece geometry.
- Workpiece Setup: Properly secure and support the workpiece to prevent vibrations and distortions during machining. Ensure the workpiece is clean and free of contaminants.
- Workpiece Inspection: Conduct pre-machining inspections to verify material quality and consistency. Check for variations in hardness or other irregularities that may affect thread quality.
Operator Training and Safety:
- Operator Training: Ensure that operators are trained in the use of thread milling equipment and programming. Continuous training helps in understanding the nuances of the process.
- Safety Precautions: Implement safety protocols to protect operators and machinery. Provide personal protective equipment (PPE) and ensure adherence to safety guidelines.
- Tool Handling: Instruct operators in proper tool handling and maintenance procedures. Mishandling tools can lead to accidents and damage.
- Emergency Procedures: Establish clear emergency procedures in case of tool breakage or other unexpected events. Quick response can minimize downtime and damage.
Incorporating these best practices and tips into your thread milling operations can significantly enhance productivity, reduce downtime, and improve thread quality. Additionally, proactive troubleshooting and problem-solving will help maintain a smooth and efficient machining process, ensuring the successful production of precision threaded components.
In Conclusion
Thread milling is a versatile and highly efficient machining process that offers numerous advantages for creating threads in a wide range of materials and applications. This comprehensive article has explored the fundamental principles and various types of thread mills, shedding light on their design, applications, and benefits.
We began by understanding the significance of threads in engineering and manufacturing, delving into the types of threads, including internal and external threads, as well as standard thread profiles like the Unified Thread Standard (UTS) and the Metric Thread Standard. We also explored traditional thread production methods, such as tapping and single-point threading, and introduced the concept of thread milling, highlighting its historical evolution and the role of CNC machining in modern thread milling.
The article then delved into the different types of thread mills, including single-form thread mills, multi-form thread mills, solid carbide thread mills, and indexable thread mills. Each type offers unique advantages and capabilities, catering to a wide array of threading requirements and materials.
Thread milling principles were thoroughly examined, covering critical aspects like tool geometry, cutter paths, speeds and feeds, and programming. Understanding these principles is crucial for achieving precise and accurate threads, optimizing tool performance, and minimizing common machining challenges.
The advantages of thread milling were explored in depth, emphasizing precision and accuracy, versatility in materials, cost-efficiency, and the ability to handle complex threads and special profiles. Thread milling’s ability to consistently deliver high-quality threads while reducing material waste and operating costs makes it an attractive choice for manufacturers across various industries.
Lastly, the article addressed troubleshooting common thread milling problems and provided best practices and tips for mitigating issues related to chip evacuation, tool breakage and wear, thread distortion, and size variation. Proper tool selection, maintenance, accurate programming, and operator training were highlighted as key elements for successful thread milling operations.
In summary, thread milling has revolutionized the process of creating threads, offering a modern, efficient, and precise alternative to traditional threading methods. By mastering the principles and best practices outlined in this article, manufacturers can harness the full potential of thread milling, improving product quality, reducing costs, and gaining a competitive edge in the ever-evolving world of engineering and manufacturing.