In the world of advanced manufacturing, precision is key, and engineers and researchers continually seek innovative methods to achieve the highest levels of accuracy and efficiency. One such cutting-edge technology that has transformed the landscape of material removal and machining processes is Electron Beam Machining (EBM). In this comprehensive guide, we will delve into the intricacies of EBM, exploring its principles, applications, advantages, challenges, and the future potential of this remarkable technology.
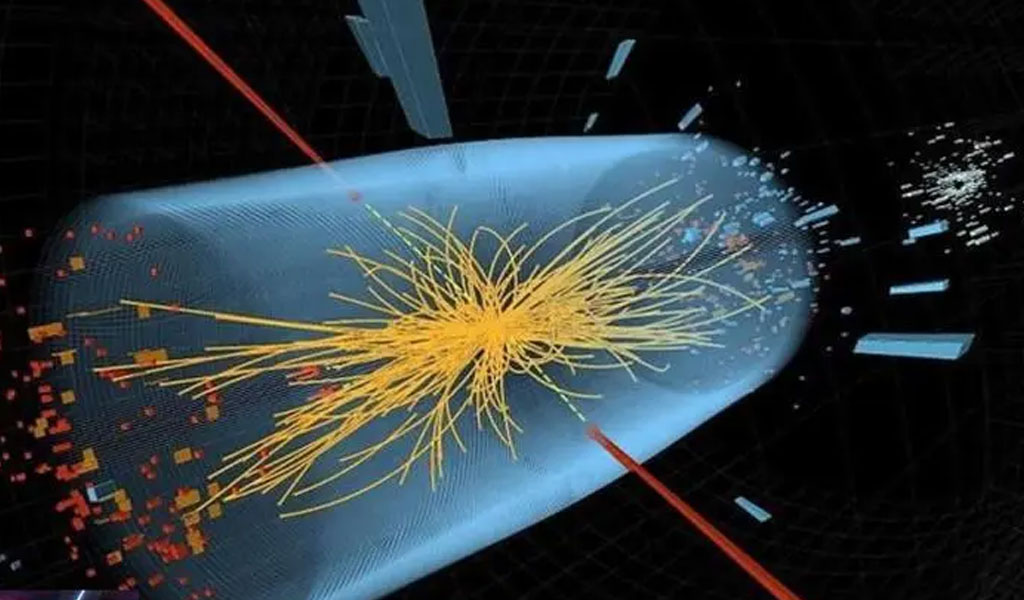
The Define Of Electron Beam Machining
Electron Beam Machining (EBM) is a non-conventional and highly precise machining process used in various industries to remove material from a workpiece. Unlike traditional machining methods that rely on mechanical cutting tools, EBM operates on the principle of controlled electron beams. These beams, composed of highly accelerated electrons, are focused on a specific spot on the workpiece, causing localized heating and vaporization of the material. This focused energy beam enables intricate and precise material removal.
The Core Components of EBM
Before delving deeper into the process, it’s essential to understand the key components that make EBM possible:
- Electron Gun: The heart of the EBM system, the electron gun generates a stream of high-energy electrons. This electron beam can be precisely controlled in terms of current and voltage.
- Magnetic Lenses: Magnetic lenses are used to focus and steer the electron beam. They ensure the electron beam’s accuracy and control over the machining process.
- Workpiece Holder: The workpiece holder secures the workpiece in place and provides the necessary adjustments for precise positioning.
- Vacuum Chamber: EBM takes place within a vacuum chamber to ensure that electrons can travel unimpeded by air molecules, making it possible to maintain precision and control over the electron beam.
- Control System: The control system, often computer-based, manages the electron beam’s parameters, such as beam current, acceleration voltage, and focus, to achieve the desired machining results.
History and Evolution of Electron Beam Machining
The history of Electron Beam Machining dates back to the early 20th century, when scientists began to explore the fascinating properties of electron beams. The development of EBM as a practical machining technique, however, took time to materialize. Here are some key milestones and the individuals and institutions that contributed to its evolution:
Early Discoveries of Electron Beam Properties
- J.J. Thomson (1897): The discovery of the electron by J.J. Thomson laid the foundation for understanding the behavior of electrons. This was a crucial step toward harnessing electron beams for practical applications.
- Vladimir Zworykin (1928): The invention of the electron microscope by Zworykin was a significant step, as it demonstrated the ability to focus and control electron beams for imaging purposes.
World War II and Advancements in EBM
RCA Laboratories: During World War II, RCA Laboratories made substantial progress in electron beam technology for military applications. They developed electron beam welding and cutting techniques that later influenced EBM’s industrial applications.
Industrial Adoption
- 1960s: EBM gained recognition as an industrial machining method, especially in the aerospace and defense sectors. The high precision and ability to work with exotic materials made EBM a valuable tool in these industries.
- 1970s to 1980s: Advancements in electronics and computer control systems led to more sophisticated and precise EBM machines. The technology became increasingly popular in various high-precision applications.
- 21st Century: EBM continues to evolve with advancements in automation, materials science, and process control, allowing for even finer levels of precision and efficiency.
Pioneering Institutions
Several institutions played a pivotal role in the development and popularization of EBM. Notable among them are the National Aeronautics and Space Administration (NASA) and the European Space Agency (ESA). These organizations have relied on EBM for manufacturing critical components used in space missions, highlighting the technology’s precision and reliability.
The Working Principles of Electron Beam Machining
The heart of Electron Beam Machining (EBM) lies in the generation of a focused, high-energy electron beam. This electron beam is the tool that interacts with the workpiece material to achieve precise material removal. Let’s delve into the intricacies of electron beam generation.
The Electron Gun
The electron gun is the central component responsible for creating and controlling the electron beam. It operates based on the principle of thermionic emission. Here’s how it works:
- Thermionic Emission: The electron gun consists of a heated filament, often made of tungsten, which emits electrons when heated to high temperatures. This emission is a result of thermionic emission, where electrons break free from the surface of the heated filament.
- Electron Acceleration: Once emitted, the electrons are accelerated by an electric field generated by an anode. This field accelerates the electrons to high velocities.
- Electron Beam Formation: The combination of the heated filament, the emitted electrons, and the electric field results in the formation of a focused electron beam. The intensity of the electron beam can be precisely controlled by adjusting the current and voltage applied to the electron gun.
Magnetic Lenses
Magnetic lenses play a crucial role in EBM by focusing and steering the electron beam. These lenses use electromagnetic fields to manipulate the path of the electrons, ensuring that they remain tightly focused on the desired spot on the workpiece. The magnetic lenses are designed to be adjustable, allowing for fine control over the electron beam’s position and size.
Material Interaction
The core principle of EBM is the interaction between the high-energy electron beam and the workpiece material. This interaction leads to localized heating, melting, and vaporization of the material, ultimately resulting in precise material removal.
Localized Heating
As the high-energy electrons impact the workpiece, they transfer their kinetic energy to the atoms or molecules in the material. This sudden infusion of energy leads to rapid heating in the small area where the electron beam is focused.
Melting and Vaporization
Once the localized temperature reaches or exceeds the melting point of the material, it begins to melt. In cases where the energy input is even higher, the material may vaporize directly from the solid state. This phase change is fundamental to the material removal process in EBM.
Energy Transfer
The energy of the electrons is significantly reduced as they interact with the material. The high energy density of the electron beam allows for material removal while maintaining precision. The shallow penetration depth of the electron beam ensures that only a minimal volume of material is affected.
Resolidification
As the electron beam moves across the workpiece, it leaves behind a path of molten or vaporized material. Once the beam is withdrawn, the material rapidly resolidifies, creating a precise cut or groove. This quick resolidification minimizes the heat-affected zone (HAZ) and contributes to the exceptional precision of EBM.
Control System
An integral part of the EBM process is the control system, typically computer-based. This system plays a critical role in managing and fine-tuning the parameters of the electron beam. Key parameters include:
- Beam Current: The amount of current supplied to the electron gun determines the number of electrons emitted, affecting the beam’s intensity.
- Acceleration Voltage: The voltage applied to the electron gun controls the speed at which electrons are accelerated, impacting their kinetic energy.
- Beam Focus: Precise control over the beam’s focus is essential for achieving the desired level of accuracy. The control system adjusts the magnetic lenses to focus the electron beam at the desired spot on the workpiece.
- Beam Positioning: The control system also allows for the precise positioning of the electron beam, enabling complex machining patterns and designs.
The Role of the Vacuum Chamber
One critical aspect of EBM is the requirement for a vacuum chamber. The vacuum chamber serves several purposes:
- Removing Air Molecules: In a vacuum, air molecules are removed, allowing the electron beam to travel unimpeded. This is essential for maintaining control and precision during the machining process.
- Preventing Oxidation and Contamination: The absence of oxygen and other gases in the vacuum chamber helps prevent oxidation and contamination of the workpiece material, especially in applications involving reactive materials.
- Ensuring Safe Operation: Operating in a vacuum reduces the risk of electrical arcing or other unwanted interactions between the electron beam and surrounding gases.
We’ve explored the fundamental principles of Electron Beam Machining. The generation of the high-energy electron beam, its interaction with the workpiece material, and the role of the control system have been detailed. Additionally, we’ve highlighted the significance of the vacuum chamber in maintaining the precision and control essential to the EBM process.
In the following chapters, we will delve into the wide range of applications where EBM plays a pivotal role and explore the various techniques and variations of this technology.
Applications of Electron Beam Machining
Electron Beam Machining (EBM) has found its place in a wide range of industries where precision, accuracy, and the ability to work with diverse materials are paramount. In this chapter, we will explore some of the key applications of EBM.
Aerospace and Defense
Precision Components for Aircraft and Spacecraft
EBM is extensively utilized in the aerospace and defense sectors for the manufacturing of critical components. The exceptional precision and minimal heat-affected zone of EBM make it ideal for crafting intricate parts like turbine blades, nozzles, and engine components. These components must withstand extreme conditions, and EBM ensures they meet the stringent quality and performance standards.
Weight Reduction and Fuel Efficiency
Aerospace manufacturers leverage EBM to reduce weight without compromising strength. By creating lightweight structures, aircraft and spacecraft can achieve better fuel efficiency and enhanced overall performance. Complex internal channels and cavities, which are challenging to produce with conventional machining methods, can be easily achieved with EBM.
Medical Device Manufacturing
Biocompatible Implants and Surgical Instruments
The medical industry benefits from EBM’s ability to work with biocompatible materials. EBM is employed in the creation of customized implants, such as orthopedic implants, dental prosthetics, and cranial plates. These implants are tailored to a patient’s specific anatomy, offering improved fit and functionality. Additionally, EBM is used in crafting high-precision surgical instruments for minimally invasive procedures.
Advanced Medical Imaging Equipment
EBM plays a role in manufacturing components for advanced medical imaging devices like X-ray tubes and electron microscopes. These devices require precision at the nanoscale, making EBM an essential technology in ensuring accurate and high-resolution imaging.
Electronics and Semiconductor Industry
Cutting and Shaping Semiconductor Materials
The electronics and semiconductor industry relies on EBM for shaping and modifying semiconductor materials like silicon wafers. EBM is capable of creating intricate microstructures and patterns on these materials, contributing to the production of advanced microchips and electronic components.
Precision Cutting of Ceramic Substrates
Ceramics are widely used in the electronics industry due to their excellent thermal and electrical properties. EBM is employed to achieve precision cutting and shaping of ceramic substrates used in electronic devices, ensuring high reliability and performance.
Automotive Sector
Lightweighting and Improved Fuel Efficiency
The automotive industry benefits from EBM by enabling the production of lightweight components. Lighter vehicles consume less fuel and emit fewer greenhouse gases. EBM is used to create lightweight structures, such as engine components, exhaust systems, and chassis parts, contributing to improved fuel efficiency.
High-Performance Engine Components
EBM’s precision capabilities are invaluable in crafting high-performance engine components, such as turbocharger blades and exhaust manifolds. These parts must withstand extreme temperatures and pressures while maintaining optimal aerodynamics. EBM ensures their integrity and performance.
Research and Development
Materials Research
EBM is an essential tool in materials research and development. Researchers use EBM to study the properties of various materials and their response to high-energy electron beams. This research aids in the development of new materials with unique characteristics and applications.
Prototyping for Innovative Applications
EBM facilitates rapid prototyping, allowing researchers and engineers to create prototypes of innovative products and components. This is particularly valuable in emerging fields like aerospace, medical devices, and electronics, where novel materials and designs are constantly evolving.
Other Applications
Beyond these major sectors, EBM has niche applications in fields like the jewelry industry for creating intricate designs in precious metals, in the production of microelectronics, and in the creation of microfluidic devices for biomedical research.
We have explored the diverse and vital applications of Electron Beam Machining. Its precision, ability to work with various materials, and minimal heat-affected zone make EBM an invaluable tool in industries where exacting standards are the norm. The technology continues to evolve, expanding its applications and playing an integral role in modern manufacturing and research.
In the following chapters, we will delve into the advantages and challenges of EBM, as well as specialized techniques and variations within this technology.
Advantages and Disadvantages of Electron Beam Machining
Advantages of EBM
Electron Beam Machining (EBM) offers a range of advantages that make it a preferred choice in various industries. These advantages are a testament to the precision and versatility of the technology:
Exceptional Precision
EBM is renowned for its unmatched precision. It can create features and cuts on a microscopic scale, making it indispensable for applications that demand extreme accuracy. This level of precision is particularly valuable in industries like aerospace, medical device manufacturing, and microelectronics.
Minimal Heat-Affected Zone (HAZ)
One of the key advantages of EBM is its ability to minimize the heat-affected zone (HAZ). The HAZ is the region of the workpiece that experiences changes in material properties due to heat generated during machining. In EBM, the rapid localized heating and cooling result in a negligible HAZ, reducing the risk of thermal distortion or damage to surrounding material.
Wide Material Compatibility
EBM is known for its ability to work with a broad spectrum of materials, from metals like titanium and stainless steel to ceramics, composites, and even refractory materials. This versatility allows EBM to serve various industries with different material requirements.
Non-Contact Process
Unlike traditional machining methods that involve physical contact between cutting tools and the workpiece, EBM is a non-contact process. This absence of contact eliminates wear and tear on cutting tools, resulting in longer tool life and reduced maintenance costs.
High Energy Density
EBM offers a high energy concentration at the point of contact. This characteristic allows for rapid material removal, resulting in high machining speeds. The focused energy beam effectively vaporizes or melts material, ensuring efficient material removal.
Challenges of EBM
While EBM offers numerous advantages, it is not without its challenges and limitations. Understanding these challenges is essential to making informed decisions about its application:
Limited Depth of Cut
One significant limitation of EBM is its limited depth of cut. EBM excels at shallow cuts and intricate detailing, but it is less effective for deep machining. The electron beam’s energy cannot penetrate deeply into the material, making it unsuitable for operations requiring substantial material removal.
Surface Finish Issues
Achieving a high-quality surface finish with EBM can be challenging. The rapid melting and resolidification of the material can result in surface irregularities and roughness. Additional post-processing steps may be necessary to achieve the desired surface quality, adding time and cost to the machining process.
Vacuum Requirements
EBM operates within a vacuum chamber, which is essential to eliminate air molecules that could impede the electron beam’s path. The vacuum chamber adds complexity to the machining setup and requires additional maintenance and safety measures.
Energy Consumption
The generation of high-energy electron beams in EBM requires a significant amount of electrical energy. This can contribute to high operational costs, particularly in industries with extensive EBM usage. Energy efficiency measures and cost considerations are necessary when implementing EBM technology.
Initial Equipment Cost
The initial investment in EBM equipment can be substantial. The cost of electron guns, magnetic lenses, vacuum chambers, and control systems can be a barrier to entry for some organizations. However, this cost is often justified by the technology’s precision and performance benefits in specific applications.
Balancing Advantages and Challenges
In summary, EBM offers a remarkable combination of precision, versatility, and the ability to work with a wide range of materials. Its advantages are particularly evident in industries where precision and minimal heat-affected zones are critical. However, EBM is not a one-size-fits-all solution and has limitations, including restricted depth of cut, surface finish issues, and energy consumption concerns.
To fully leverage the advantages of EBM while mitigating its challenges, it is essential to carefully consider the suitability of the technology for a specific application and implement appropriate strategies to address its limitations. As EBM technology continues to evolve, ongoing research and innovation may pave the way for further improvements, potentially reducing some of the current limitations.
EBM Techniques and Variants
Electron Beam Machining (EBM) is a versatile technology with various specialized techniques and variants designed to meet specific manufacturing and research needs. In this chapter, we will explore some of the key EBM techniques and their applications.
Electron Beam Welding (EBW)
Precision Welding
Electron Beam Welding (EBW) is a technique that uses a high-energy electron beam to join metal parts with exceptional precision. This process offers advantages such as deep penetration, minimal heat-affected zones, and the ability to weld dissimilar metals. EBW is commonly used in the aerospace, automotive, and nuclear industries for applications like joining turbine blades and nuclear reactor components.
Aerospace Applications
In the aerospace sector, EBW is particularly valuable for joining critical components like engine parts, airframe structures, and fuel tanks. The process ensures welds with high integrity, reducing the risk of defects and enhancing the structural reliability of aerospace components.
Electron Beam Melting (EBM)
Additive Manufacturing
Electron Beam Melting (EBM) is a form of additive manufacturing, often referred to as 3D printing. In EBM, a high-energy electron beam is used to selectively melt and solidify metal powders, layer by layer, to build intricate 3D structures. This technique is especially useful for creating complex and lightweight components in industries such as aerospace, healthcare, and automotive.
Customized Medical Implants
EBM is widely used in the production of customized medical implants. Its ability to work with biocompatible materials, combined with the precision of additive manufacturing, allows for the creation of patient-specific implants. These implants can be tailored to fit an individual’s unique anatomy, improving patient outcomes and reducing the risk of implant rejection.
Electron Beam Drilling (EBD)
Precision Hole Drilling
Electron Beam Drilling (EBD) is a specialized technique used for precise hole drilling in various materials, including metals, ceramics, and composites. EBD employs a high-energy electron beam to remove material in a controlled and accurate manner, making it suitable for applications that demand high-quality holes with tight tolerances.
Microelectronics and Aerospace
EBD finds applications in the microelectronics industry for drilling tiny holes in semiconductor materials. In the aerospace sector, it is used for creating precision holes in engine components and turbine blades, where maintaining the material’s structural integrity is critical.
Electron Beam Additive Manufacturing (EBAM)
Metal Powder Consolidation
Electron Beam Additive Manufacturing (EBAM) is an advanced technique used for metal powder consolidation. It involves melting and solidifying metal powders layer by layer to create complex, high-strength parts. EBAM offers the advantage of rapid manufacturing of large-scale components with reduced material waste.
Aerospace and Defense
EBAM is embraced by the aerospace and defense industries for producing large, intricate structural components, such as aircraft wings, fuselages, and missile components. Its ability to create lightweight yet robust parts contributes to improved fuel efficiency and performance.
Electron Beam Surface Treatment
Surface Modification
Electron Beam Surface Treatment involves using an electron beam to modify the surface properties of materials without altering their bulk properties. This technique is employed for surface hardening, alloying, and improving wear resistance in components. It is especially useful in industries where component longevity is crucial.
Automotive and Tooling
In the automotive industry, EBM surface treatment is used to enhance the wear resistance of engine components and gears, extending their service life. In tool and die manufacturing, it is applied to improve the durability and performance of cutting tools.
Electron Beam Microfabrication
Nanoscale Precision
Electron Beam Microfabrication is a specialized variant of EBM that focuses on achieving nanoscale precision. It is used for creating intricate microstructures and patterns on a variety of materials, including semiconductors and advanced optical components.
Semiconductor Manufacturing
In the semiconductor industry, Electron Beam Microfabrication is essential for producing microelectronics with ever-decreasing feature sizes. It plays a vital role in the production of integrated circuits and microelectromechanical systems (MEMS).
We have explored various specialized techniques and variants of Electron Beam Machining. These adaptations of the core EBM technology cater to specific requirements in industries ranging from aerospace and healthcare to automotive and microelectronics. The versatility and precision of EBM continue to drive innovation in these sectors, allowing for the creation of advanced and customized components and structures. As technology evolves, it is likely that we will witness further refinements and applications of EBM techniques.
Electron Beam Machining vs. Laser Beam Machining
Electron Beam Machining (EBM) and Laser Beam Machining (LBM) are two distinct advanced machining processes used in manufacturing and material removal. They differ in their principles, energy sources, applications, and some key characteristics. Here’s a comparison of Electron Beam Machining and Laser Beam Machining:
Energy Source:
- EBM: Electron Beam Machining uses a high-velocity beam of electrons, generated by a focused electron gun. These high-energy electrons are directed towards the workpiece.
- LBM: Laser Beam Machining utilizes a high-intensity, coherent beam of light generated by a laser source, typically a laser diode, fiber, or CO2 laser.
Energy Type:
- EBM: Electron beam machining relies on kinetic energy, as high-speed electrons are used to bombard and remove material from the workpiece.
- LBM: Laser beam machining relies on the thermal energy of the laser beam to melt, vaporize, or ablate the material.
Penetration Depth:
- EBM: Electron beams can penetrate deep into the material, making it suitable for thick and dense materials.
- LBM: Laser beams have limited penetration depth, mainly effective for surface or shallow material removal.
Material Compatibility:
- EBM: Electron beam machining is versatile and can be used for a wide range of materials, including metals, ceramics, and composites.
- LBM: Laser beam machining is suitable for a variety of materials, but it is especially effective on materials with high absorption coefficients for the laser wavelength, such as metals and some plastics.
Precision:
- EBM: EBM is known for its high precision and fine detail capabilities due to the small electron beam size.
- LBM: Laser machining offers high precision as well, but it may not achieve the same level of detail as EBM in some cases.
Heat Generation:
- EBM: EBM generates minimal heat during the machining process, which is an advantage for materials sensitive to heat, like some polymers.
- LBM: Laser machining can generate significant heat, which can potentially cause thermal damage to the workpiece or affect the surrounding area.
Operating Environment:
- EBM: EBM typically requires a vacuum environment to prevent electron scattering, making it more complex to set up and operate.
- LBM: Laser machining can be performed in a regular atmosphere, which is more convenient and versatile.
Speed:
- EBM: EBM can have relatively slower material removal rates compared to some laser machining processes.
- LBM: Laser machining processes can be faster for certain applications, especially when focused on material ablation.
Applications:
- EBM: EBM is often used for precision drilling, welding, and cutting of complex shapes in materials like aerospace alloys and ceramics.
- LBM: LBM is used in a wide range of applications, including engraving, cutting, welding, and marking on materials like metals, plastics, and semiconductors.
Both EBM and LBM have their own advantages and limitations, and the choice between them depends on the specific requirements of a given manufacturing or material processing task.
Similarities:
Attribute | Electron Beam Machining (EBM) | Laser Beam Machining (LBM) |
---|---|---|
Energy Source | Focused beam of energy particles | Focused beam of coherent light (laser) |
Precision | Exceptional precision | High precision |
Material Compatibility | Wide material compatibility | Wide material compatibility |
No Thermal Contact | Non-contact process | Non-contact process |
Versatility | Various applications in different industries | Versatile for cutting, welding, marking |
Differences:
Attribute | Electron Beam Machining (EBM) | Laser Beam Machining (LBM) |
---|---|---|
Depth of Cut | Limited depth of cut | Deeper penetration compared to EBM |
Heat-Affected Zone | Minimal heat-affected zone | Larger heat-affected zone |
Equipment Requirements | Requires a vacuum chamber for operation | No need for a vacuum chamber |
Energy Density | High-energy density | Moderately lower energy density compared |
to EBM | ||
Surface Finish | Surface finish may require additional | Typically good surface finish, but |
post-processing for smoother results | quality may vary depending on parameters | |
Initial Equipment Cost | Initial equipment cost can be relatively | Initial equipment cost may be lower |
high due to electron gun and vacuum chamber | compared to EBM | |
Energy Consumption | Higher energy consumption due to electron | Typically lower energy consumption due |
beam generation | to laser source efficiency |
In Conclusion
Electron Beam Machining (EBM) is a precision manufacturing technology that has emerged as a pivotal player in various industries, pushing the boundaries of what is achievable in terms of precision, material compatibility, and versatility. As we conclude our journey through the world of EBM, we recap the key takeaways and the significance of this technology.
The Power of Precision
EBM stands out for its exceptional precision. With the ability to focus high-energy electrons to a minute spot, it can achieve intricate and microscopic cuts and shapes with unmatched accuracy. This level of precision is indispensable in industries like aerospace, medical devices, and microelectronics, where exacting standards are a prerequisite.
Versatility Across Materials
One of EBM’s key strengths is its ability to work with a wide range of materials. From metals to ceramics, composites, and refractory materials, EBM demonstrates its versatility. This makes it an invaluable tool in industries where diverse materials need to be precisely machined.
Minimizing Heat-Affected Zones
EBM’s capacity to minimize the heat-affected zone (HAZ) is a game-changer. The small HAZ reduces the risk of thermal distortion and damage to surrounding material, a critical advantage in applications where material integrity is paramount.
Challenging the Status Quo
EBM challenges conventional manufacturing methods by offering an alternative approach. It doesn’t rely on mechanical cutting tools, offering a non-contact process with extended tool life. This shift in methodology has implications for various industries, including aerospace, defense, healthcare, and microelectronics.
Balancing Advantages and Challenges
While EBM boasts a range of advantages, it is not without its limitations. These include a limited depth of cut, surface finish challenges, vacuum chamber requirements, and energy consumption concerns. Recognizing and addressing these limitations are essential for effective EBM implementation.
Ongoing Innovation
EBM continues to evolve with advancements in automation, materials science, and process control. As technology progresses, we can expect improvements in energy efficiency, cost-effectiveness, and the expansion of EBM applications into new industries.
The Future of Precision Manufacturing
In conclusion, Electron Beam Machining is a driving force in the world of precision manufacturing. Its applications are vast, from crafting components for space missions to manufacturing customized medical implants. The technology’s remarkable precision, material compatibility, and minimal heat-affected zones have propelled it to the forefront of modern manufacturing.
As EBM technology advances, it is likely to find new and innovative applications. The journey of EBM reflects the relentless pursuit of precision and excellence in manufacturing, with the potential to revolutionize industries and contribute to the development of cutting-edge products and research.
In an era where precision and innovation are paramount, EBM stands as a beacon of possibilities and a testament to human ingenuity in the world of machining and manufacturing. As the journey continues, we can only anticipate more remarkable developments and applications for this remarkable technology.