In the world of electrical engineering, wire gauge plays a crucial role in determining the appropriate size of a wire for a specific application. One of the widely used standards in this regard is the American Wire Gauge (AWG) system. This system is fundamental in ensuring the safe and efficient transmission of electrical power across various applications, ranging from household electrical wiring to industrial and commercial projects. In this comprehensive guide, we will delve deep into the concept of wire gauge, explore the intricacies of the American Wire Gauge system, discuss its significance, and provide detailed insights into the calculations involved.
View More Articles:
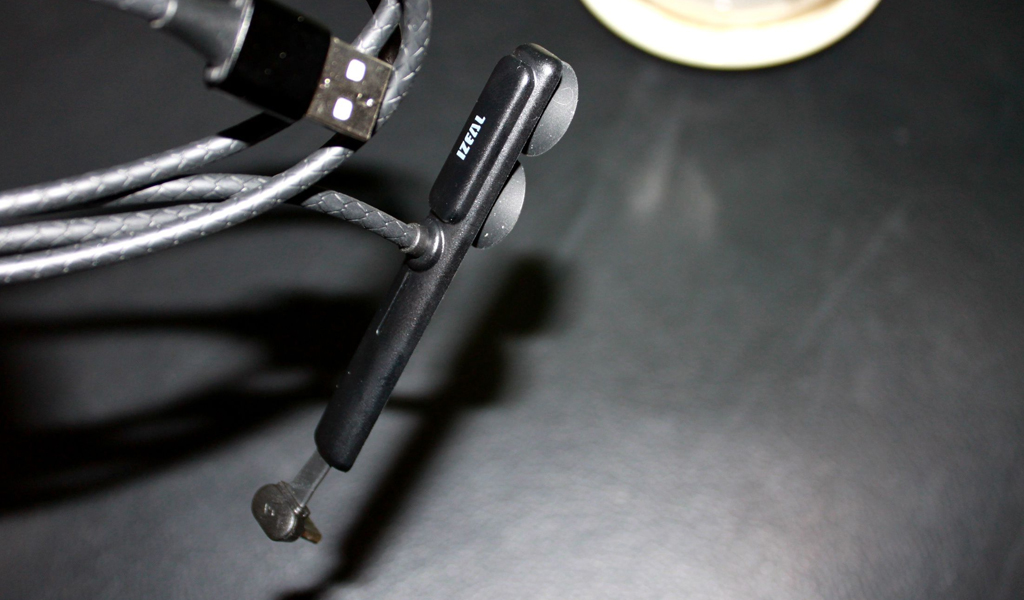
What Is Wire Gauge
Wire gauge, in simple terms, is a measure of a wire’s diameter or thickness. It is typically expressed as a numerical value and is essential for a variety of reasons, including safety, efficiency, and performance in electrical systems. This measurement plays a crucial role in determining a wire’s electrical and thermal characteristics, such as its resistance, current-carrying capacity, and the amount of power it can safely transmit.
At its core, wire gauge is a system of classification that assigns a specific number to a wire’s size. This number is inversely related to the wire’s diameter, which means that as the gauge number increases, the wire’s diameter decreases. For instance, a wire with a lower gauge number is thicker and can carry more current than a wire with a higher gauge number, which is thinner.
The Importance of Wire Gauge
Wire gauge is of paramount importance in a multitude of applications where electrical wiring is involved. Whether you are wiring a house, designing an electronic circuit, installing car audio, or working on industrial machinery, understanding wire gauge is vital for several key reasons:
- Safety: Using the appropriate wire gauge ensures that the wire can safely carry the expected current without overheating or causing a fire hazard. Undersized wires can overheat, leading to dangerous situations.
- Efficiency: Properly sized wires minimize power losses due to resistance. This is especially critical in long-distance power transmission or in applications where energy efficiency is a priority.
- Performance: In electronic circuits, using the right wire gauge can prevent signal degradation and ensure optimal performance. It’s crucial in applications where precise signal transmission is required, such as data communication and audio systems.
- Compliance with Codes and Standards: In many regions, electrical codes and standards dictate the use of specific wire gauges for different applications. Adhering to these regulations is not just a matter of legality but also a matter of safety and reliability.
- Cost-Efficiency: Using the correct wire gauge helps avoid overspending on unnecessarily thick wires. It ensures that you use the right-sized wires for the task at hand, optimizing material and installation costs.
As we delve deeper into the world of wire gauge, we’ll explore its historical development, the intricacies of the American Wire Gauge system, and its practical applications in various fields, providing you with a comprehensive understanding of this fundamental concept.
Historical Development of Wire Gauges
Wire gauges have a rich history that spans centuries, evolving in response to the ever-increasing demands of electrical systems and technology. The development of wire gauges can be traced back to early sizing systems, which laid the foundation for the more standardized systems in use today. Understanding this historical context provides insight into the significance of modern wire gauge systems like the American Wire Gauge (AWG).
1.Early Wire Sizing Systems
Before the advent of standardized wire gauges, wire sizing was a rather inconsistent affair, often relying on ad-hoc methods. Craftsmen and engineers measured wire diameters using various tools and recorded the sizes according to their preferences. These early systems lacked uniformity and precision, which presented challenges in ensuring the reliability and safety of electrical systems.
One of the earliest systems was the Birmingham Wire Gauge (BWG), which emerged in the 19th century in the United Kingdom. It measured wire diameters based on increments, but there was no standardization beyond this concept, making it difficult for engineers and electricians to communicate effectively about wire sizes.
The development of wire sizing systems was crucial due to the growing need for consistent and standardized wire diameters, especially as electricity became more prevalent and played a more significant role in daily life. The lack of standardization led to inefficiencies, inconsistencies in electrical systems, and even safety hazards.
2.The Emergence of American Wire Gauge (AWG)
The American Wire Gauge (AWG), also known as the Brown & Sharpe wire gauge, emerged as a response to the challenges posed by the absence of a standardized wire sizing system. This system, developed in the United States in the mid-19th century, brought much-needed order to the world of wire sizing.
The AWG system is based on a mathematical formula that assigns a specific number to each wire size. In this system, a lower AWG number corresponds to a thicker wire, while a higher number signifies a thinner wire. This numeric representation of wire sizes not only facilitated communication but also introduced precision to the field of electrical engineering and wiring.
The development of the AWG system was a collaborative effort, with various engineers, including Joseph Rogers Brown and Lucian Sharpe, contributing to its standardization. The primary goal was to create a reliable system that could be consistently applied across industries, ensuring safety and efficiency in electrical systems.
The AWG system gained popularity not only in the United States but also internationally. Its widespread adoption marked a significant turning point in the history of wire gauges, providing a standardized, easy-to-understand method for determining wire size. This, in turn, paved the way for safer and more reliable electrical systems, from household wiring to industrial applications.
Understanding the American Wire Gauge (AWG)
The American Wire Gauge (AWG) system is a crucial standard for determining the size of electrical wires. It provides a uniform and easily understandable way to categorize wires based on their diameter. This section will delve into the details of the AWG system, including how it compares to other wire sizing systems and its key characteristics.
AWG vs. SWG and Other Systems
While the American Wire Gauge (AWG) is widely recognized in the United States and many parts of the world, it’s essential to acknowledge that other wire sizing systems exist, each with its own historical and regional significance. Here, we will primarily focus on the comparison between AWG and the British Standard Wire Gauge (SWG) as well as touch briefly on other systems.
1. AWG vs. SWG:
- American Wire Gauge (AWG): As mentioned earlier, the AWG system is widely used in the United States. It assigns a numerical value to wire sizes, where a lower number represents a thicker wire. The AWG system is prevalent in North America and is recognized as the primary wire sizing standard for most applications, including household wiring, automotive wiring, and electronics.
- Standard Wire Gauge (SWG): The Standard Wire Gauge system, also known as the Imperial Wire Gauge, was developed in the United Kingdom. While it is not as widely used as AWG, it remains significant in British and Commonwealth countries. The key difference between SWG and AWG lies in the sizing increments and the specific sizes assigned to each gauge number. The SWG system may have different values for wire sizes compared to AWG.
2. Other Wire Sizing Systems:
- Circular Mil System: The Circular Mil System, primarily used in the United States, quantifies wire size based on circular mils. One circular mil is equal to the area of a circle with a diameter of one mil (one-thousandth of an inch). This system is often employed in the calculation of wire resistance.
- European Wire Gauge (EWG): The European Wire Gauge system is used in Europe and some other regions. Similar to AWG, it assigns a numerical value to wire sizes, but it uses different sizing increments and may have distinct sizes for each gauge number.
- Japanese Industrial Standards (JIS): Japan uses its own wire sizing standards, such as the JIS C 3102 standard, which differs from AWG in its gauge values and increment steps. It’s important to be aware of these standards when working with Japanese-made electrical components.
While there are regional variations in wire sizing systems, the American Wire Gauge (AWG) is one of the most widely accepted and recognized systems worldwide. It’s critical for professionals in various industries to be aware of these different systems, especially if they work in a global context or with components manufactured in different regions.
Key Characteristics of AWG
The American Wire Gauge (AWG) system possesses several key characteristics that make it a fundamental and indispensable tool in the field of electrical engineering and related industries:
- Numeric Representation: AWG assigns a specific number to each wire size, which simplifies communication and ensures a clear understanding of the wire’s diameter.
- Inverse Relationship: In the AWG system, as the gauge number decreases, the wire diameter increases. This inverse relationship is a critical aspect of the system and helps in easy identification of wire thickness.
- Uniform Sizing Increments: AWG provides consistent sizing increments, making it easier to select the appropriate wire size for a given application. Each step in gauge number corresponds to a specific change in wire diameter.
- Cross-Sectional Area: AWG can be used to calculate the cross-sectional area of a wire, which is essential for determining current-carrying capacity and resistance.
- Industry Standard: AWG is the standard wire sizing system in the United States and is widely accepted in many other countries, ensuring consistency in electrical and electronic systems.
Wire Gauge Chart
A wire gauge chart, often referred to as an American Wire Gauge (AWG) chart, is a valuable reference tool in the world of electrical engineering and electronics. It presents a comprehensive list of wire sizes along with corresponding gauge numbers and important information. In this section, we will examine the structure and significance of the AWG chart.
Analyzing the AWG Chart Table
The AWG chart is typically presented in a tabular format, with rows and columns. Here’s a breakdown of how to interpret the information in an AWG chart:
- Gauge Number (AWG): The leftmost column of the chart lists the gauge numbers, starting from the thickest wire at the top (e.g., 0000 or 4/0 AWG) and progressing to the thinnest wire at the bottom (e.g., 40 AWG). Each gauge number corresponds to a specific wire diameter.
- Diameter (Inches and Millimeters): The chart provides columns for wire diameters in both inches and millimeters. This allows users to quickly identify the diameter of a wire based on its gauge number.
- Circular Mil Area: The circular mil area represents the cross-sectional area of the wire in circular mils (a unit of area used in the electrical industry). This value is helpful for calculating wire resistance and current-carrying capacity.
- Resistivity (Ohms per Circular Mil Foot): This column provides the resistance of the wire material in ohms per circular mil foot. The resistivity value is crucial for calculating the resistance of a specific length of wire.
- Amps for Chassis Wiring: This column specifies the maximum current-carrying capacity for wires used in chassis wiring, which typically refers to wiring within an enclosed piece of equipment, like a computer or an amplifier.
- Amps for Power Transmission: This column indicates the maximum current a wire can carry for power transmission applications, such as long-distance power lines.
Practical Use of the AWG Chart Table
The AWG chart is an invaluable resource for engineers, electricians, and anyone working with electrical and electronic systems. Here are some practical ways in which the AWG chart is used:
- Selecting the Right Wire Size: When designing an electrical or electronic system, the chart helps you determine the appropriate wire size based on the current-carrying requirements of the application. This ensures that the wire can carry the necessary current without overheating.
- Calculating Resistance: The chart, along with resistivity values, is used to calculate the resistance of a specific length of wire. This is essential for understanding power losses and voltage drop in a circuit.
- Sizing Circuit Breakers and Fuses: Engineers use the chart to size circuit breakers and fuses, ensuring they provide protection for the wire and connected devices.
- Wire Selection for Specific Applications: Different applications require wires of varying sizes. The chart is consulted to select the appropriate wire for household wiring, automotive applications, low-voltage electronics, and more.
- Estimating Current-Carrying Capacity: By referring to the chart, you can estimate the current-carrying capacity of a wire, helping ensure safety and code compliance in electrical installations.
- Comparing Different Sizing Systems: The AWG chart is also used to compare wire sizes across different systems, such as AWG and SWG, to ensure compatibility in global or cross-regional projects.
- Troubleshooting and Maintenance: In maintenance and troubleshooting scenarios, the chart can be used to identify the wire size and characteristics of existing systems, aiding in repairs and replacements.
The AWG chart is a versatile tool that simplifies the process of selecting the right wire size, understanding wire characteristics, and ensuring the safety and efficiency of electrical and electronic systems. It is a fundamental reference for professionals and enthusiasts working in the field of electrical engineering and related industries.
Wire Diameter and Cross-Sectional Area
Wire diameter and cross-sectional area are fundamental characteristics of wires that are crucial for various applications in electrical engineering and electronics. Understanding how to calculate wire diameter and determine cross-sectional area is essential for selecting the right wire size and assessing its electrical properties.
Calculating Wire Diameter
Wire diameter is a measure of the thickness of a wire, typically expressed in inches or millimeters. It’s a critical parameter when choosing the right wire for a specific application. The wire diameter can be calculated using the following formulas:
For Circular Wires:
- In Inches: Diameter (in inches) = √[ (Diameter (in circular mils)) / 1000 ]
- In Millimeters: Diameter (in millimeters) = √[ (Diameter (in circular mils)) / 1973.5 ]
For Non-Circular Wires (e.g., rectangular or square wires):
For non-circular wires, the calculation of the diameter is based on the wire’s cross-sectional area and its shape. You will need to measure the dimensions of the wire’s cross-section and then calculate the equivalent circular diameter.
For example, if you have a wire with a circular mil area of 50,000 circular mils, you can use the formula above to calculate its diameter in either inches or millimeters.
Determining Cross-Sectional Area
The cross-sectional area of a wire is the total area of the wire’s transverse cut. It’s a crucial parameter for determining a wire’s current-carrying capacity, as well as for calculating resistance. The cross-sectional area is typically expressed in circular mils, square millimeters, or square inches, depending on the system being used.
To calculate the cross-sectional area of a wire, you can use the following formulas:
For Circular Wires:
- In Circular Mils: Cross-Sectional Area (in circular mils) = (Diameter (in mils))^2
- In Square Millimeters: Cross-Sectional Area (in square millimeters) = π * (Diameter (in millimeters) / 2)^2
- In Square Inches: Cross-Sectional Area (in square inches) = π * (Diameter (in inches) / 2)^2
For Non-Circular Wires (e.g., rectangular or square wires):
The cross-sectional area of non-circular wires is calculated based on the dimensions of the wire’s cross-section. For a rectangular wire, the cross-sectional area would be the product of its width and thickness in the appropriate units (e.g., square millimeters or square inches).
The calculated cross-sectional area is a key parameter for determining the wire’s current-carrying capacity and resistance, as it directly affects these electrical characteristics. It is also vital for selecting wires that can handle the required current without overheating or experiencing excessive voltage drop.
Resistance and Current-Carrying Capacity
Resistance is a fundamental electrical property of wires and plays a crucial role in determining their current-carrying capacity. Understanding how resistance and current-carrying capacity are related is essential for designing safe and efficient electrical systems.
Ohm’s Law and Wire Resistance
Ohm’s Law, named after the German physicist Georg Simon Ohm, describes the relationship between voltage (V), current (I), and resistance (R) in an electrical circuit. Ohm’s Law is expressed by the formula:
V=I*R
- V (Voltage): The potential difference or electrical pressure that drives current through a wire, measured in volts (V).
- I (Current): The flow of electric charge, measured in amperes (A), which represents the rate at which charge moves through a wire.
- R (Resistance): The opposition to the flow of current, measured in ohms (Ω).
In the context of wire gauge and sizing, resistance is a critical parameter that depends on the wire’s dimensions (diameter and length) and the material from which it is made. The longer and thinner a wire is, the higher its resistance, while a shorter and thicker wire has lower resistance. The resistance of a wire can be calculated using the formula:
R=(ρ*L)/A
- R (Resistance): The resistance of the wire in ohms (Ω).
- ρ (Resistivity): A material-specific property representing how strongly a given material opposes the flow of electric current, measured in ohm-meter (Ω·m).
- L (Length): The length of the wire in meters (m).
- A (Cross-Sectional Area): The cross-sectional area of the wire in square meters (m²).
The cross-sectional area of the wire, which we discussed in the previous section, is essential in the resistance calculation. A wire with a larger cross-sectional area will have lower resistance, while a smaller cross-sectional area leads to higher resistance.
As an example, when selecting a wire for a specific application, it’s essential to consider the expected current and the allowable voltage drop. If the wire’s resistance is too high for the desired current, it can lead to power losses and a drop in voltage that affects the performance of the connected devices. Therefore, choosing the appropriate wire gauge, which determines the wire’s cross-sectional area, is vital to minimize resistance and optimize the electrical system’s efficiency.
Current-Carrying Capacity of Wires
The current-carrying capacity of a wire, also known as ampacity, refers to the maximum amount of current a wire can carry safely without overheating. This capacity depends on various factors, including the wire’s size, material, insulation, and the environment in which it is installed.
When selecting a wire for a specific application, it’s crucial to consider the current-carrying capacity to prevent overheating, which can lead to safety hazards and damage to the wire. Factors that affect the current-carrying capacity include:
- Wire Size: Larger wires, with a greater cross-sectional area, can carry more current without overheating. Therefore, selecting an appropriate wire gauge is essential.
- Wire Material: Different materials, such as copper and aluminum, have varying conductivity and resistance properties, influencing a wire’s ampacity.
- Ambient Temperature: The temperature of the surrounding environment can impact a wire’s ability to dissipate heat. High temperatures can reduce the current-carrying capacity, while lower temperatures can increase it.
- Insulation Type: The type of insulation used on the wire can affect heat dissipation. Some insulating materials have better thermal properties than others.
- Conduit and Installation Method: The manner in which the wire is installed, such as whether it is placed in a conduit or exposed to open air, can influence its ability to dissipate heat.
Engineers and electricians use standardized tables and guidelines to determine the current-carrying capacity of wires in different scenarios. These guidelines ensure that wires are selected and installed to meet safety standards and prevent overheating or fire hazards in electrical systems. It’s essential to consult these tables and adhere to local electrical codes when designing and installing electrical circuits.
Wire Materials and Their Impact on Gauge
The choice of wire material is a critical consideration when determining wire gauge for electrical and electronic applications. Different materials have varying properties, including electrical conductivity, thermal characteristics, and resistance to environmental factors. In this section, we’ll explore the impact of materials, with a focus on copper, aluminum, and other conductive materials, on wire gauge selection.
Copper Wire
Copper is one of the most common materials used for electrical wiring due to its excellent electrical conductivity. It’s highly ductile, making it easy to draw into wires of various sizes, and it’s resistant to corrosion, making it suitable for both indoor and outdoor applications.
The American Wire Gauge (AWG) system is predominantly used for copper wire sizing in the United States. Copper wire is available in a wide range of gauges, from very thick (lower AWG numbers) to very thin (higher AWG numbers). The high electrical conductivity of copper allows for smaller wire gauges to carry more current, making it a popular choice for many applications.
When selecting copper wire for an application, the key factors to consider include the required current-carrying capacity, voltage drop limitations, and the wire’s resistance. Thicker copper wires (lower AWG numbers) can handle higher currents with less voltage drop and reduced resistance, while thinner wires (higher AWG numbers) are used for low-current and low-voltage applications.
Aluminum Wire
Aluminum is another commonly used material for electrical wiring, particularly in applications that require lightweight conductors. Aluminum is lighter than copper and, when used in large-scale applications like power transmission lines, can significantly reduce material and installation costs.
However, aluminum has some distinct differences compared to copper, which impact wire gauge selection:
- Conductivity: Aluminum has lower electrical conductivity than copper, which means it has higher resistance for a given wire size. As a result, aluminum wires need to be larger in cross-sectional area (lower AWG numbers) to carry the same current as a copper wire.
- Thermal Expansion: Aluminum has a higher coefficient of thermal expansion compared to copper. This means aluminum wires may experience more significant changes in length when exposed to temperature variations, potentially leading to loosening connections if not properly accounted for.
- Oxidation and Corrosion: Aluminum is more susceptible to oxidation and corrosion compared to copper. This can impact the longevity and performance of aluminum wire, especially in outdoor or corrosive environments.
- Compatibility: Connections between aluminum and copper wires require special connectors and joint compounds to prevent galvanic corrosion, making it more complex to work with both materials in the same system.
Other Conductive Materials
While copper and aluminum are the most prevalent conductive materials in electrical applications, there are other materials used for specific purposes:
- Silver: Silver is the most conductive metal but is rarely used for wiring due to its high cost. It’s primarily employed in specialized applications, such as high-frequency electronics.
- Gold: Gold offers excellent conductivity and corrosion resistance. It’s used in precision electronics and connectors, where reliability and low resistance are critical.
- Tin: Tin-plated copper wire combines the conductivity of copper with the corrosion resistance of tin. It is often used in automotive and marine applications.
Conductivity is a measure of a material’s ability to conduct electric current. It’s the reciprocal of resistance. Materials with high conductivity have low resistance, and materials with low conductivity have high resistance. The conductive properties of a material impact the choice of wire gauge for a given application. Lower-resistance wires, such as those made of copper, are selected for high-current applications, while higher-resistance wires may be used for lower-current or specialized applications.
The choice of wire material, whether it’s copper, aluminum, or another conductive material, significantly influences wire gauge selection. Electrical engineers and electricians must carefully consider the material’s conductivity, resistance, and other properties when designing and installing electrical systems to ensure safety, efficiency, and compliance with industry standards.
Wire Gauge Calculations
Selecting the right wire gauge for your application is a critical step in designing an electrical or electronic system. It involves considering factors such as the required current-carrying capacity, voltage drop limitations, safety, and compliance with electrical codes and standards.
Selecting the Right Wire for Your Application
Choosing the appropriate wire gauge for your application is essential for ensuring safety and performance. Here are the steps to help you select the right wire gauge:
- Determine the Current Requirements: Calculate or estimate the maximum current that the wire will need to carry in your application. This depends on the devices or loads connected to the wire.
- Consider Voltage Drop: Depending on your application, you may have limitations on voltage drop. Excessive voltage drop can affect the performance of connected devices. Calculate the maximum allowable voltage drop for your application.
- Consult Applicable Codes and Standards: Review local electrical codes and standards to ensure compliance. Many codes specify minimum wire sizes based on factors such as current, circuit type, and environmental conditions.
- Calculate the Resistance: Use the formula for wire resistance (R = ρ * L / A), where ρ is the resistivity of the wire material, L is the wire length, and A is the wire’s cross-sectional area. Calculate the resistance for the chosen wire gauge and the length of wire in your system.
- Compare Resistance and Current-Carrying Capacity: Ensure that the calculated resistance is within acceptable limits for your application. The wire should be able to carry the required current without excessive power losses or overheating.
- Account for Temperature: Consider the operating temperature of the wire and any potential temperature changes. Different wire insulations and environments may affect the wire’s temperature rating.
- Size Up if in Doubt: If you are uncertain about the wire gauge selection, it’s generally safer to choose a larger (lower AWG number) wire gauge than to risk using an undersized wire. This provides a margin of safety and reduces the likelihood of overheating.
Voltage Drop and Power Loss Considerations
Voltage drop is an important consideration in electrical and electronic systems. It refers to the decrease in voltage as current flows through a wire due to its resistance. Excessive voltage drop can lead to performance issues in connected devices and, in extreme cases, may violate electrical codes. To manage voltage drop:
- Calculate the voltage drop using Ohm’s Law (V = I * R), where V is voltage drop, I is current, and R is the resistance of the wire.
- Compare the calculated voltage drop to the allowable voltage drop for your application. If the drop is too high, consider using a larger wire gauge to reduce resistance.
- In cases where voltage drop is critical, especially in long-distance power transmission or low-voltage applications, use voltage drop calculators or tables to select the appropriate wire gauge.
Power loss, often in the form of heat generated by wire resistance, can be a concern in electrical systems. Excessive power loss can lead to inefficiency and can even pose safety risks. To manage power loss:
- Calculate the power loss in the wire using the formula P = I^2 * R, where P is power loss, I is current, and R is resistance.
- Select a wire gauge that keeps power loss within acceptable limits for your application.
Safety and Code Compliance
Safety is paramount when selecting a wire gauge. Choosing the right wire size ensures that wires do not overheat, potentially causing fires or damage to equipment. It also helps maintain the proper voltage levels for the operation of electrical devices.
Compliance with local electrical codes and standards is a legal and safety requirement. These codes often specify minimum wire sizes based on the type of circuit, the expected current, the location of installation, and other factors. Failure to comply with these codes can result in safety hazards, fines, or legal consequences.
When selecting a wire gauge, always consider safety and compliance. It’s advisable to work with qualified professionals, such as electricians or electrical engineers, to ensure that your wire gauge selection aligns with safety regulations and industry standards. Additionally, consult local authorities and electrical inspectors to verify compliance with applicable electrical codes in your region.
In Conclusion
In the complex world of electrical engineering, electronics, and the myriad applications that rely on the seamless transmission of electrical power, wire gauge plays a central and often underappreciated role. It is the silent conductor that ensures the smooth flow of electricity, connecting our modern world in ways we often take for granted. This comprehensive article has shed light on the crucial importance of wire gauge, especially the widely used American Wire Gauge (AWG) system, and its historical development, applications, and key characteristics.
From its origins in a world of inconsistent wire sizing systems to the emergence of the AWG system, wire gauge has come a long way. It has evolved to provide engineers, electricians, and electronics enthusiasts with a standardized, easy-to-understand method for determining wire sizes and ensuring safety, efficiency, and compliance with codes and standards.
We’ve explored how to interpret wire gauge charts, analyze the AWG chart table, and practically apply it to select the right wire for various applications. Understanding wire diameter and cross-sectional area is critical for calculating resistance and current-carrying capacity, helping us design systems that deliver power reliably and safely.
Resistance and current-carrying capacity are pivotal aspects of wire gauge calculations. Ohm’s Law serves as the foundation for comprehending how resistance and current are interrelated, enabling us to design electrical systems that meet the desired performance criteria.
Wire materials, such as copper and aluminum, have a profound impact on wire gauge selection. The electrical conductivity, resistance, thermal characteristics, and environmental considerations of these materials influence the choice of wire for specific applications.
In the final sections, we discussed how to select the right wire for your application, considering factors such as current requirements, voltage drop, safety, and compliance with codes and standards. Voltage drop and power loss are important considerations, as they affect the performance and efficiency of electrical systems.
Safety and code compliance should never be overlooked. Adhering to local electrical codes and standards is not only a legal requirement but also a fundamental aspect of ensuring safety in electrical installations.
The Central Role of Wire Gauge
Wire gauge may be an often-overlooked element of electrical and electronic systems, but it holds a central role in the reliability, safety, and efficiency of these systems. It is the foundation upon which electrical circuits are built, the conduit through which power flows, and the assurance of safety in a world powered by electricity.
Whether in a household, an automotive application, an industrial facility, or a data center, wire gauge is the unifying thread that connects and powers our modern world. By understanding its significance and applying the principles and calculations presented in this article, we empower ourselves to make informed decisions, design efficient systems, and uphold the highest standards of safety and performance.
How to Stay Informed and Updated
The world of electrical engineering, electronics, and related technologies is ever-evolving. To stay informed and updated on wire gauge and related topics, consider the following strategies:
- Continuing Education: Enroll in courses, workshops, or online programs focused on electrical engineering and related subjects. This can help you stay current with the latest developments in wire gauge and other pertinent areas.
- Professional Associations: Join professional organizations such as the Institute of Electrical and Electronics Engineers (IEEE) or the National Electrical Contractors Association (NECA). These organizations often provide access to industry publications, conferences, and networking opportunities.
- Industry Publications: Subscribe to industry magazines, journals, and newsletters that cover electrical engineering and electronics. These publications frequently feature articles, case studies, and updates on wire gauge and related topics.
- Online Forums and Communities: Participate in online forums and communities dedicated to electrical engineering and electronics. These platforms are excellent for discussing challenges, sharing knowledge, and staying updated on industry trends.
- Manufacturer Resources: Consult wire and cable manufacturers’ websites for technical resources, product specifications, and guides on wire gauge selection.
- Local Electrical Codes: Stay informed about updates to local electrical codes and standards. Attend seminars or workshops related to electrical code changes to ensure compliance in your area.
- Research and Academia: Follow the latest research and academic advancements in electrical engineering and materials science, as these fields often contribute to improvements in wire materials and sizing systems.
By staying informed and up-to-date, you can continue to make informed decisions, improve your skills, and contribute to the safety and efficiency of electrical and electronic systems. The ever-advancing world of technology and engineering requires a commitment to lifelong learning and adaptation.