Flatness and parallelism are two critical concepts in engineering and manufacturing that play a fundamental role in ensuring precision and quality in various applications. Despite their similar connotations, they denote distinct aspects of surface geometry and orientation. This article delves into the intricate differences between flatness and parallelism, discussing their definitions, applications, challenges, and significance in modern industries. It highlights the essential role of these concepts in ensuring the functionality and integrity of mechanical components, emphasizing their relevance in fields such as aerospace, automotive, and precision engineering. By providing a comprehensive analysis of their implications and practical implementation, this article aims to offer readers a comprehensive understanding of the crucial role that flatness and parallelism play in shaping the contemporary landscape of engineering and manufacturing.
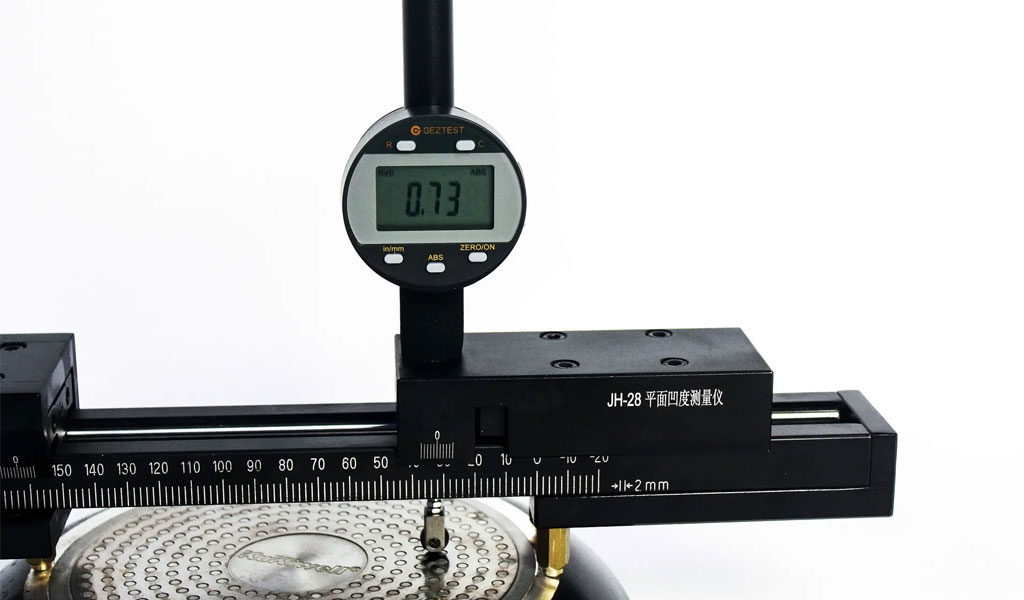
What Is Flatness
In the realm of engineering and manufacturing, precision and accuracy are paramount. The quality and functionality of mechanical components, from critical aerospace parts to everyday consumer products, depend heavily on the concept of flatness. This fundamental parameter ensures that surfaces are perfectly even or level, free from deviations or irregularities that could compromise performance and reliability. In this section, we will delve into the concept of flatness, exploring its definition, measurement techniques, and its paramount importance in various industries.
Flatness, as a geometric characteristic, refers to the degree to which a surface conforms to a perfectly even plane. It implies that the surface, when measured against a reference plane, exhibits minimal height variations across its entire area. In simpler terms, a flat surface is one where any two points on that surface are at the same height or parallel to each other. This concept is defined by a specific tolerance or deviation from the ideal flat plane, typically expressed in units such as micrometers (µm) or inches (in).
The Type Of Flatness
In the context of engineering and manufacturing, there are different types or categories of flatness specifications and assessments, each with specific characteristics and applications. These types of flatness are often defined based on the geometric features or surfaces under consideration and the requirements of the given application. Here are some common types of flatness:
Total Indicator Reading (TIR) Flatness
Plate Flatness
Surface Flatness
Warp Flatness
Global Flatness vs. Local Flatness
Free State vs. Clamped State Flatness
While flatness focuses on deviations from an ideal flat plane, roughness deals with the fine details of the surface texture, including peaks and valleys. Both parameters are essential for determining the overall quality of a surface but address different aspects of its geometry.
Engineering flatness refers to the design specifications and requirements set by engineers to ensure that a component meets its functional and performance criteria. Manufacturing flatness relates to the actual measurement and assessment of flatness during the production process, ensuring that components meet the specified engineering flatness standards.
The type of flatness considered in a given application depends on the industry, the specific component or surface under scrutiny, and the functional requirements. Choosing the appropriate type of flatness is crucial for ensuring that components perform as intended and meet the necessary quality standards.
Measurement of Flatness
Measuring flatness accurately is essential to ensure that components meet the required standards. Various measurement techniques and tools are employed to quantify flatness, each with its own strengths and suitability for different applications:
- Coordinate Measuring Machines (CMMs): CMMs are precision instruments used to measure the 3D coordinates of points on a surface. They can assess flatness by comparing the surface profile to a predefined reference plane. Advanced CMMs can provide highly accurate flatness measurements.
- Optical Profilometers: These non-contact instruments use light to capture detailed 3D surface profiles. Optical profilometry is useful for measuring the flatness of components with delicate or highly reflective surfaces.
- Laser Scanners: Laser scanning technology employs laser beams to capture the 3D geometry of a surface. It is quick and accurate, making it suitable for assessing flatness in a range of applications.
- Surface Plates: Surface plates are precision-ground, flat reference surfaces used as a benchmark for measuring flatness. Engineers place the component to be assessed on the surface plate and measure deviations using various methods, such as dial indicators or gauge blocks.
- Interferometers: Interferometers use the interference of light waves to measure the variations in a surface. They are incredibly precise but are often used for research or high-precision applications.
Importance of Flatness
Flatness is a critical parameter in various industries for the following reasons:
- Interchangeability and Compatibility: In applications where components need to fit together or slide against each other, such as in machinery and automotive systems, flatness is crucial. Deviations from flatness can lead to misalignments, increased friction, or even component failure.
- Sealing and Gasket Performance: In industries like aerospace and plumbing, ensuring that mating surfaces are perfectly flat is vital for creating effective seals. Any deviations can result in leaks and compromise the integrity of the system.
- Surface Finish: In the manufacturing of products like optical lenses, mirrors, and electronic displays, maintaining precise flatness is essential for achieving the desired surface finish and optical quality.
- Precision Measurements: In metrology and measurement instruments, flat reference surfaces are critical for achieving highly accurate measurements and calibrations. Any distortion in these surfaces can introduce errors in measurements.
- Material Removal Processes: During processes like grinding, lapping, or polishing, flatness is a crucial parameter to ensure that material is removed uniformly and efficiently, achieving the desired surface finish.
- Electronics Manufacturing: The flatness of substrates and printed circuit boards (PCBs) is essential for the proper functioning of electronic components. Flatness ensures that soldered connections are uniform and reliable.
- Aerospace and Aviation: The aerospace industry demands stringent flatness requirements to guarantee the structural integrity and performance of aircraft components. Deviations from flatness can affect aerodynamics and safety.
- Semiconductor Manufacturing: In the semiconductor industry, the flatness of silicon wafers is crucial. Any unevenness can lead to defects in integrated circuits, affecting device performance.
Flatness is a fundamental concept in engineering and manufacturing, with far-reaching implications for product performance, quality, and safety. Precise measurement and control of flatness are essential for ensuring that components meet stringent standards and operate as intended, making it an indispensable parameter in numerous industries.
What Is Parallelism
Parallelism is a fundamental geometric concept that plays a pivotal role in ensuring precise alignment and functionality of components and systems in various engineering and manufacturing applications.
In this section, we will delve into the concept of parallelism, explore its core principles, examine its diverse applications, and address the challenges associated with maintaining parallelism in real-world scenarios.
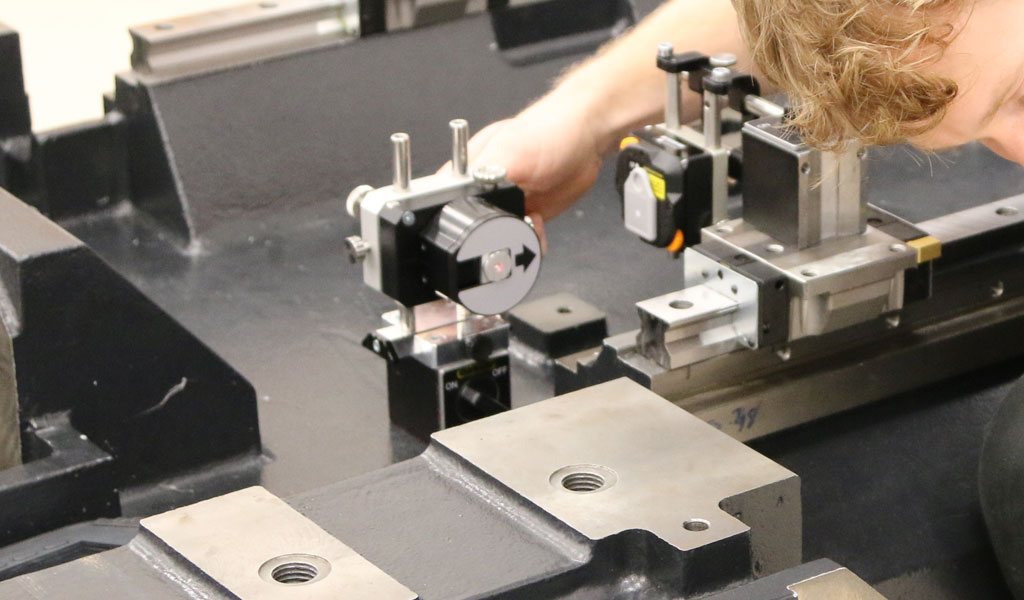
Parallelism refers to the state in which two or more lines, surfaces, or features remain equidistant at all points, maintaining a consistent separation distance. In simpler terms, parallelism ensures that objects or features are perfectly aligned and do not deviate from a predetermined reference plane or axis.
The Type Of Parallelism
Parallelism is a fundamental geometric concept, and its types often depend on the specific applications and the nature of the parallel elements being considered. Here are some common types of parallelism:
Line Parallelism
Surface Parallelism
Axis Parallelism
Planar Parallelism
Angular Parallelism
Surface Parallelism vs. Axis Parallelism
Intercomponent parallelism assesses the parallelism between different components or parts of a larger assembly. Intra-component parallelism, on the other hand, focuses on ensuring that multiple elements within a single component, such as the surfaces of a machine bed or the blades of a turbine, remain parallel to each other.
Free state parallelism measures the parallelism of components or elements when they are not constrained or clamped. Clamped state parallelism evaluates parallelism when components are secured or clamped in place, which can affect their alignment.
In geometric dimensioning and tolerancing (GD&T), parallelism can be specified with various tolerance zones, such as a parallelism zone or a parallelism modifier. These GD&T specifications define the allowable deviation from perfect parallelism, and they can be applied to lines, surfaces, or axes.
The choice of parallelism type depends on the specific requirements of the application and the industry. Maintaining the correct type of parallelism is critical for ensuring that components and systems operate efficiently, safely, and in accordance with the necessary quality standards.
Core Principles of Parallelism
- Equidistance: The fundamental principle of parallelism is that all elements involved remain at the same distance from each other. This equidistance can apply to lines, surfaces, or other geometrical features. For example, in the context of two parallel lines, the distance between them remains constant along their entire length.
- Consistency: Parallelism demands that the relationship between elements remains consistent. If two surfaces or lines are initially parallel, they should stay parallel, and any deviations are considered non-parallelism.
- Reference Plane or Axis: Parallelism is often defined with respect to a reference plane or axis. Deviations from this reference are measured and evaluated to determine the degree of parallelism.
Applications of Parallelism
Parallelism is a critical concept with diverse applications in engineering and manufacturing across various industries:
- Shafts and Bearings: In mechanical systems, the parallelism of shafts and the alignment of bearing surfaces are essential for reducing friction, ensuring smooth rotation, and preventing premature wear.
- Gears and Gear Trains: The proper alignment and parallelism of gears are crucial for efficient power transmission and noise reduction in gear systems.
- Railway Tracks: Ensuring parallelism in railway tracks is vital for the safe and stable movement of trains. Deviations from parallelism can lead to derailments.
- Optical Systems: In optics, the parallelism of lenses and mirrors is crucial for maintaining image quality and preventing optical aberrations.
- Automotive Components: Parallelism is important in various automotive applications, such as aligning wheels, axles, and suspension components to ensure vehicle stability and handling.
- Structural Engineering: In construction, maintaining parallelism in beams, columns, and other structural elements is essential for the stability and integrity of buildings and bridges.
- Printers and Plotters: Parallelism plays a role in the precise movement of print heads and paper in printers and plotters, ensuring accurate printing and plotting.
- Machining and Manufacturing: The alignment of machine tool components, such as the spindle and workpiece, is crucial for precision machining operations.
Challenges in Maintaining Parallelism
While parallelism is a fundamental concept, achieving and maintaining it can be challenging in real-world scenarios:
- Manufacturing Tolerances: Variations in material properties, machining processes, and assembly can introduce deviations from ideal parallelism. Manufacturers must establish and adhere to strict tolerances to control these deviations.
- Thermal Effects: Temperature changes can cause components to expand or contract, potentially affecting parallelism. Compensating for thermal effects or employing temperature-stable materials can be necessary.
- Wear and Tear: Over time, components may experience wear, leading to changes in parallelism. Regular maintenance and replacement may be needed to restore proper alignment.
- Complex Assemblies: In intricate systems with multiple components, achieving and maintaining parallelism among all elements can be particularly challenging. Proper design, precision machining, and assembly techniques are essential.
- Environmental Factors: Vibrations, shocks, and other environmental factors can impact parallelism. Implementing shock-absorbing or vibration-dampening solutions can help mitigate these effects.
- Testing and Measurement: Accurately measuring and assessing parallelism can be challenging. The use of precision measuring equipment and techniques is essential for quality control.
- Cost and Complexity: Achieving high levels of parallelism often requires precision manufacturing and testing, which can be more expensive and complex compared to less demanding specifications.
In conclusion, parallelism is a fundamental concept with wide-ranging applications in engineering and manufacturing. It ensures the alignment and stability of critical components and systems, contributing to overall performance and safety. However, maintaining parallelism comes with its set of challenges, requiring careful consideration of factors like manufacturing tolerances, environmental conditions, and material properties to ensure consistent alignment and functionality.
Differentiating Flatness and Parallelism
In the world of engineering and manufacturing, precision and accuracy are indispensable. Two core concepts that underpin these qualities are flatness and parallelism. Although these terms are often used interchangeably or confused with one another due to their apparent similarity, they represent distinct geometric characteristics with unique applications and implications. In this section, we will comprehensively differentiate flatness and parallelism, shedding light on their definitions, measurement methods, and practical significance in various industries.
Definitions:
- Flatness:Flatness, as the name suggests, pertains to the evenness or levelness of a surface. It is the characteristic of a surface that is perfectly smooth and free from deviations or irregularities. In essence, a flat surface, when measured against a reference plane, exhibits minimal height variations across its entire area. Deviations from this ideal flat state are quantified within specified tolerance limits. The primary concern with flatness is ensuring that a surface conforms to a designated reference plane and maintains a consistent height throughout its extent.
- Parallelism:Parallelism, on the other hand, refers to the state in which two or more lines, surfaces, or features remain equidistant at all points, maintaining a consistent separation distance. Parallelism does not necessarily concern the flatness of individual surfaces but rather the alignment and uniformity of the relationships between multiple features or components. In essence, it ensures that these features do not deviate from a predetermined reference plane or axis and maintain a constant separation, or in simpler terms, that they remain “parallel” to each other.
Measurement:
- Flatness:Flatness is typically measured by evaluating the surface profile of a component concerning a reference plane. Various measurement tools, such as Coordinate Measuring Machines (CMMs), optical profilometers, laser scanners, and surface plates, are employed to assess and quantify flatness. These tools help in determining the height deviations across the entire surface, allowing for the quantification of flatness based on predefined tolerances.
- Parallelism:Parallelism, on the other hand, is assessed by measuring the separation or alignment between two or more features, lines, or surfaces relative to a reference plane or axis. Instruments like height gauges, dial indicators, and laser interferometers are often used to measure parallelism. The focus here is on determining the consistent distance or alignment between the elements, rather than the individual flatness of those elements.
Practical Significance:
- Flatness:The practical significance of flatness primarily lies in its impact on how a single surface interacts with other components. Flatness is crucial in applications where components need to fit together, slide against each other, or maintain precise sealing. Deviations from flatness can lead to misalignments, increased friction, and compromised functionality. Applications of flatness include aerospace components, automotive gasket seals, precision engineering, and optical systems.
- Parallelism:Parallelism is critical in ensuring that multiple components or features align correctly. It is particularly important in applications where precise orientation and consistent separation are required, such as in shafts, gears, bearings, and structural elements. Deviations from parallelism can lead to inefficient power transmission, misalignment, and structural instability. Industries where parallelism is essential include automotive (axles, wheels), manufacturing (machining setups), construction (structural beams), and optics (lens alignment).
While flatness and parallelism may both involve the geometric characteristics of surfaces and components, they have distinct purposes and applications. Flatness focuses on the individual evenness of a surface relative to a reference plane, ensuring minimal height deviations. Parallelism, in contrast, concentrates on the consistent alignment and separation of multiple features, ensuring they remain equidistant from each other. Understanding these differences is essential for selecting the appropriate measurement techniques and quality control measures to achieve the desired precision and functionality in various engineering and manufacturing processes.
The Significance of Flatness and Parallelism
The concepts of flatness and parallelism, though seemingly straightforward, underpin the foundations of precision and quality in engineering and manufacturing. In this concluding section, we emphasize the significance of flatness and parallelism in these domains and how they collectively contribute to the functionality, safety, and reliability of a wide range of products and systems.
- Precision and Quality Assurance:Flatness and parallelism serve as fundamental benchmarks for achieving precision and quality in engineering and manufacturing. By maintaining strict adherence to specified tolerances for these characteristics, industries can produce components and systems that perform reliably and consistently. This precision is particularly critical in applications where even slight deviations can lead to a domino effect of problems, including misalignments, friction, and premature wear.
- Mechanical Integrity:In industries where safety and mechanical integrity are paramount, such as aerospace and automotive manufacturing, the importance of flatness and parallelism cannot be overstated. Deviations from flatness can affect the performance of gasket seals and mating surfaces, potentially leading to catastrophic failures. Parallelism is essential for maintaining the alignment of crucial components like gears, bearings, and axles, ensuring the safe and efficient operation of vehicles and machinery.
- Efficiency and Performance:Efficiency and performance gains are realized when flatness and parallelism are carefully maintained. In applications like semiconductor manufacturing and optics, flatness ensures the precision and quality of products. Parallelism in machinery and mechanical systems optimizes power transmission, reduces friction, and minimizes energy losses. These gains are particularly significant in today’s competitive landscape, where every improvement in efficiency counts.
- Reliability and Longevity:The reliability and longevity of products are directly influenced by the flatness and parallelism of critical components. In structural engineering and construction, the parallelism of beams and columns determines the stability and safety of buildings. Deviations from parallelism can compromise structural integrity and lead to costly repairs or, in the worst cases, catastrophic failures. In contrast, precise parallelism ensures the durability and longevity of structures.
- Competitive Advantage:Companies that excel in maintaining flatness and parallelism standards gain a competitive advantage. Their products consistently meet or exceed customer expectations in terms of performance, quality, and reliability. This enhanced reputation for precision and quality often leads to increased market share, customer loyalty, and a strong competitive edge in the global marketplace.
- Evolving Challenges:While flatness and parallelism are timeless concepts, they are not immune to evolving challenges. As industries push the boundaries of technology, materials, and design, the need for even tighter tolerances and the development of advanced measurement and control techniques become apparent. Manufacturers must adapt to these challenges to remain at the forefront of their respective fields.
In conclusion, flatness and parallelism are not just abstract concepts in engineering and manufacturing but are real-world parameters with immense implications. They are the pillars upon which the modern world is built, ensuring that the structures we inhabit, the products we use, and the systems we rely on meet the highest standards of performance, safety, and reliability. As industries continue to advance, understanding and mastering flatness and parallelism will remain central to achieving success and maintaining the highest standards of precision and quality.