CNC machining, short for Computer Numerical Control machining, has revolutionized the manufacturing industry by automating and enhancing precision in cutting and shaping materials. Central to this technology is the cutting path, a fundamental concept that plays a pivotal role in the CNC machining process. In this comprehensive guide, we will delve deep into CNC machining cutting paths, exploring the principles, designs, and techniques that are essential for mastering this critical aspect of modern manufacturing.
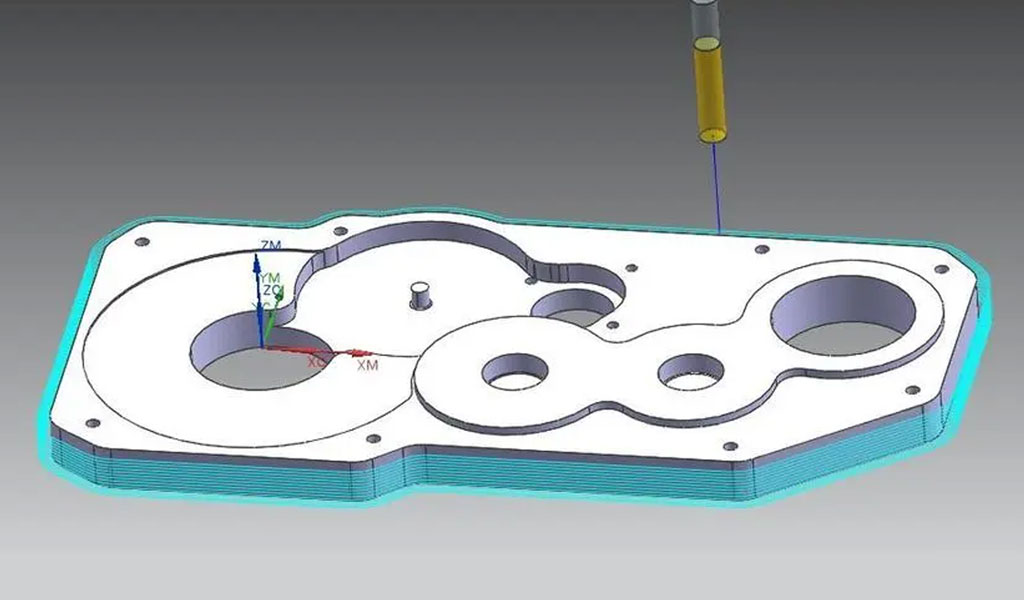
The Fundamentals of Cutting Paths
CNC machining is a sophisticated manufacturing process that relies on precise tool movements to shape materials into desired components. At the heart of this process are cutting paths, a fundamental concept that plays a critical role in achieving accuracy and efficiency in CNC machining. In this comprehensive guide, we will explore the fundamentals of cutting paths, covering their definition, importance in CNC machining, their relationship with toolpaths, coordinate systems, types of cutting paths, tool selection and geometry, cutting parameters, and the role of Computer-Aided Manufacturing (CAM) software in generating toolpaths.
What is a Cutting Path?
Definition and Importance in CNC Machining
A cutting path is the designated route or trajectory that a cutting tool follows while removing material from a workpiece during CNC machining. It serves as a roadmap for the tool, guiding it through the necessary motions to achieve the desired shape, size, and surface finish of the final product. Cutting paths are a fundamental aspect of CNC machining, influencing factors such as precision, efficiency, and cost-effectiveness.
The importance of cutting paths in CNC machining cannot be overstated:
- Precision: Accurate cutting paths are essential for achieving the correct dimensions and tolerances in the final product. Any deviation from the intended path can result in defects and inaccuracies.
- Efficiency: Well-designed cutting paths optimize tool movements, reducing unnecessary travel and minimizing machining time. This not only increases productivity but also prolongs tool life.
- Cost-Efficiency: Efficient cutting paths reduce material wastage and energy consumption, contributing to cost savings in the manufacturing process.
Relationship between Cutting Paths and Toolpaths
While cutting paths and toolpaths are closely related concepts, they are not synonymous. A toolpath encompasses a broader set of instructions that guide a CNC machine’s movements during machining. Toolpaths include information about tool changes, spindle speeds, feed rates, and more, in addition to the specific cutting path.
In essence, a cutting path represents the actual trajectory followed by the cutting tool, while a toolpath encompasses all the instructions needed to control the CNC machine to execute that cutting path. The precise generation and control of toolpaths are critical for ensuring that the machining process aligns with the intended design and material specifications.
Coordinate Systems
Cartesian, Polar, and Cylindrical Coordinate Systems
Coordinate systems are used to specify the position and movement of a cutting tool within the three-dimensional space of a CNC machine. The most common coordinate systems in CNC machining include:
- Cartesian Coordinate System: This system uses three linear axes—X, Y, and Z—to define points in space. The X-axis typically represents horizontal movement, the Y-axis vertical movement, and the Z-axis movement along the depth or height of the workpiece. The Cartesian system is the foundation of most CNC machining operations.
- Polar Coordinate System: In polar coordinates, points are defined by their radial distance from a reference point (origin) and an angular measurement relative to a reference axis. This system is often used in rotary applications, such as turning operations.
- Cylindrical Coordinate System: A combination of the Cartesian and polar systems, cylindrical coordinates introduce a third coordinate that represents height or depth (usually denoted as Z). This system is particularly useful when machining features that extend in multiple directions.
How CNC Machines Interpret Coordinates
CNC machines rely on the programmed coordinates to determine the tool’s position and movement. The machine’s controller interprets these coordinates and translates them into precise movements of the cutting tool along the designated axes. This interpretation is done using specialized software that reads and executes the CNC program, which typically consists of G-codes and M-codes.
The correct interpretation and execution of coordinates are critical for achieving accuracy and repeatability in CNC machining processes. Any errors or inconsistencies in the programming or interpretation of coordinates can result in machining defects and deviations from the desired cutting path.
Types of Cutting Paths
Contour Cutting Paths
Contour cutting paths are used to define the outer perimeter or outline of a workpiece or a specific part of it. These paths follow the contour of the design and are commonly employed in operations where precise part dimensions and shape are essential.
Pocketing and Profiling Paths
- Pocketing paths involve machining enclosed areas or pockets within the workpiece. The tool moves in a series of parallel passes, gradually removing material within the defined boundaries. Pocketing is often used to create recessed features in parts.
- Profiling paths, on the other hand, are used to create internal or external profiles, such as slots, channels, or edges. Profiling is crucial for achieving specific part geometries and dimensional accuracy.
Drilling and Hole-Making Paths
Drilling paths are employed to create holes in the workpiece, ranging from simple through-holes to more complex features like countersinks or counterbores. Hole-making paths specify the tool’s movements to create holes of various sizes and shapes.
Each type of cutting path serves a specific purpose in CNC machining, and the selection of the appropriate path depends on the part’s design and the machining operation’s requirements.
Tool Selection and Geometry
Understanding Tool Geometries
Selecting the right cutting tool and understanding its geometry are critical aspects of designing effective cutting paths. Different types of cutting tools, such as end mills, drills, reamers, and inserts, have specific geometries that determine their cutting capabilities. Some key aspects of tool geometries include:
- Number of Flutes: The number of flutes or cutting edges on a tool significantly impacts material removal rates, surface finish, and chip evacuation. Tools with more flutes tend to provide better surface finish but may require lower feed rates.
- Helix Angle: The helix angle of a tool’s flutes influences chip evacuation and cutting forces. Higher helix angles are often used for efficient chip removal, especially in materials that produce long, stringy chips.
- Rake Angle: The rake angle determines the cutting edge’s orientation concerning the workpiece. Positive rake angles promote efficient cutting, while negative rake angles are used for tougher materials.
- Edge Geometry: The shape and angle of the cutting edge impact tool performance. Common edge geometries include square, ball-nose, and corner-radius.
Tool Selection Considerations
Choosing the right tool for a CNC machining operation involves considering several factors:
- Material Type: Different materials, such as metals, plastics, ceramics, and composites, require specific tool materials and coatings to optimize tool life and performance.
- Cutting Speed: The material’s properties and hardness influence the appropriate cutting speed. Hard materials often require slower cutting speeds to prevent excessive tool wear.
- Feed Rate: Feed rate, or the rate at which the tool advances into the material, affects chip formation, chip evacuation, and the quality of the cut.
- Depth of Cut: The depth of cut determines how much material the tool removes in each pass. It affects cutting forces, tool wear, and machining time.
Selecting the right tool and considering these factors is crucial for designing effective cutting paths that meet the requirements of the specific machining operation.
Cutting Parameters
Speed, Feed Rate, and Depth of Cut
Cutting parameters play a vital role in shaping the cutting path and ultimately determining the efficiency and quality of the machining process. The primary cutting parameters include:
- Speed: Cutting speed, often referred to as surface speed, is the rate at which the cutting tool moves relative to the workpiece’s surface. It is typically measured in surface feet per minute (SFM) or meters per minute (m/min). Cutting speed affects chip formation, tool life, and heat generation during machining.
- Feed Rate: Feed rate is the rate at which the tool advances into the material, typically measured in inches per minute (IPM) or millimeters per minute (mm/min). A proper feed rate ensures efficient chip evacuation, minimizes tool wear, and maintains part quality.
- Depth of Cut: The depth of cut refers to how deep the tool penetrates into the workpiece during each pass. It influences cutting forces, chip formation, and the overall time required to complete the cutting path.
How Cutting Parameters Affect the Cutting Path
The choice of cutting parameters directly affects the cutting path and the overall machining process:
- Cutting Speed: Increasing the cutting speed can improve productivity but may require adjustments to the feed rate and depth of cut to maintain cutting efficiency and part quality. High-speed machining techniques optimize this parameter.
- Feed Rate: A higher feed rate can reduce machining time but must be balanced with cutting speed and depth of cut to prevent tool wear, overheating, and poor surface finish.
- Depth of Cut: The depth of cut determines the thickness of material removed in each pass. Adjusting this parameter can impact tool life, cutting forces, and chip evacuation.
Optimizing cutting parameters is essential to achieve the desired results, and it often involves a balance between achieving efficient material removal and maintaining tool longevity and part quality.
Toolpath Generation Software
CAM (Computer-Aided Manufacturing) Software
Computer-Aided Manufacturing (CAM) software is a critical component of CNC machining, as it translates design specifications into actionable instructions for the CNC machine. CAM software provides the following essential functions:
- Import CAD Models: CAM software can import 3D CAD models or 2D drawings of the part to be machined, ensuring accurate representation.
- Toolpath Generation: CAM software automatically generates toolpaths based on the part’s geometry and the desired machining operations. This includes selecting appropriate tooling, specifying cutting parameters, and generating toolpath trajectories.
- Optimization: CAM software optimizes toolpaths for efficiency, minimizing unnecessary tool movements and reducing machining time.
- Simulation: Many CAM packages include simulation features that allow users to visualize the machining process and detect any errors or collisions before actual machining. This helps prevent costly mistakes and ensures safe operations.
- Post-Processing: Once the toolpaths are generated, CAM software produces a G-code program that the CNC machine can understand and execute. This G-code includes instructions for tool changes, spindle speeds, and feed rates.
Popular CAM Software Options and Their Features
The choice of CAM software can significantly impact the efficiency and capabilities of CNC machining. Some popular CAM software options include:
- Autodesk Fusion 360: Fusion 360 offers a comprehensive suite of CAD/CAM tools, including 3D modeling, toolpath generation, and simulation. It is suitable for a wide range of industries and applications.
- SolidWorks CAM: Integrated with SolidWorks, this CAM software provides seamless transition from design to machining. It offers a user-friendly interface and robust toolpath generation capabilities.
- Mastercam: Mastercam is known for its advanced toolpath generation and customization options. It caters to a variety of machining applications, from 2D milling to multi-axis machining.
- Siemens NX CAM: NX CAM is part of the Siemens PLM Software suite and offers powerful CAM capabilities for complex machining tasks. It integrates well with other Siemens design and manufacturing solutions.
- HSMWorks: Integrated with Autodesk Inventor and SolidWorks, HSMWorks is known for its high-speed machining capabilities and ease of use.
The choice of CAM software should align with the specific needs of the machining operation, including the complexity of the parts to be machined, the available CNC machine capabilities, and the desired level of automation and optimization.
Understanding the fundamentals of cutting paths is essential for anyone involved in CNC machining. These paths are the guiding trajectories that ensure precision and efficiency in shaping raw materials into finished parts. By considering coordinate systems, types of cutting paths, tool selection, cutting parameters, and CAM software, manufacturers can optimize their machining processes, reduce costs, and deliver high-quality products. With the right knowledge and tools, CNC machining becomes a powerful and versatile technology capable of realizing intricate and precise designs.
Designing Effective Cutting Paths
In CNC machining, the art of designing effective cutting paths is crucial for achieving precision and efficiency in manufacturing processes. This section delves into the key aspects of designing cutting paths, including material considerations, geometric considerations, toolpath optimization, 2D and 3D machining techniques, roughing and finishing passes, and the importance of toolpath simulation and verification.
Material Considerations
How Material Properties Impact Cutting Path Design
The choice of material significantly influences cutting path design. Different materials exhibit varying hardness, ductility, thermal conductivity, and other properties that directly impact machining processes. Here’s how material properties affect cutting path design:
- Hardness: Hard materials like metals require slower cutting speeds and more robust tooling to avoid excessive tool wear. Softer materials, such as plastics, allow for higher cutting speeds.
- Thermal Conductivity: Materials with high thermal conductivity, like copper, can dissipate heat quickly, while low-conductivity materials, such as composites, tend to retain heat. This affects tool life and machining parameters.
- Brittleness: Brittle materials are prone to chipping and fracturing, especially in the presence of sharp tool movements. Careful consideration of toolpath design is necessary to prevent damage.
Best Practices for Different Materials
Designing cutting paths that account for material-specific characteristics is essential. Here are some best practices for different material types:
- Metals: Use proper cooling and lubrication to manage heat generated during machining. Implement strategies like climb milling for better surface finishes.
- Plastics: Pay attention to chip evacuation to prevent melting or burr formation. Opt for lower cutting speeds and feed rates to avoid overheating.
- Composites: Consider the orientation of fibers in composites when planning toolpaths. Specialized tooling may be required to handle the abrasive nature of composite materials.
Geometric Considerations
Strategies for Creating Complex Shapes and Contours
Designing cutting paths for intricate shapes and contours requires careful planning and consideration. Here are strategies to tackle this challenge:
- Scalability: Divide complex shapes into smaller, more manageable sections. Machining each section individually simplifies toolpath generation.
- 3D Modeling: Utilize 3D CAD models to visualize the part and create toolpaths that follow the desired contour. CAM software can automatically generate toolpaths based on the 3D model.
- Helical Toolpaths: For deep pockets or intricate contours, consider helical toolpaths that gradually spiral down, reducing abrupt direction changes and tool stress.
Avoiding Toolpath Collisions and Inefficiencies
Toolpath collisions and inefficiencies can lead to costly errors and suboptimal machining. To avoid these issues:
- Collision Detection: Use CAM software with collision detection features to identify potential tool collisions with the workpiece, fixtures, or machine components.
- Optimization Algorithms: Implement optimization algorithms that minimize unnecessary tool movements, reducing machining time and wear on the tool.
- Toolpath Smoothing: Smooth toolpaths to eliminate sharp corners and sudden changes in direction, which can lead to vibration, chatter, and poor surface finish.
Toolpath Optimization
Optimizing toolpaths is crucial for efficiency and tool longevity in CNC turn-mill machining. Here are strategies for achieving this:
Strategies for Minimizing Machining Time
Reducing machining time not only increases productivity but also lowers operational costs. To minimize machining time:
- High-Speed Machining (HSM): Utilize HSM techniques to increase cutting speeds while maintaining precision. HSM is particularly effective for removing material rapidly.
- Trochoidal Milling: Trochoidal milling employs circular toolpaths within a pocket, reducing tool wear and cutting forces.
- Adaptive Machining: Adaptive toolpaths adjust cutting conditions based on real-time data, optimizing tool engagement and minimizing machining time.
Reducing Tool Wear and Extending Tool Life
Prolonging tool life not only saves on tool replacement costs but also ensures consistent part quality. To reduce tool wear and extend tool life:
- Proper Feeds and Speeds: Use recommended cutting speeds, feed rates, and depth of cuts for the selected tool and material to avoid premature tool wear.
- Toolpath Optimization Algorithms: Employ CAM software with toolpath optimization algorithms that minimize heat generation, prevent excessive tool engagement, and distribute tool wear evenly.
- Coolant and Lubrication: Implement efficient coolant and lubrication systems to dissipate heat and reduce friction between the tool and workpiece.
2D and 3D Machining
Techniques for Creating Intricate 2D Profiles
2D machining involves the creation of flat profiles or contours on a workpiece. To achieve intricate 2D profiles:
- Toolpath Order: Plan the sequence of toolpaths carefully to minimize material repositioning and optimize chip evacuation.
- Multiple Depths: When machining deeper profiles, consider employing multiple passes at varying depths to reduce tool stress and ensure accuracy.
- Ramp and Plunge Techniques: Use ramping and plunging strategies to enter and exit the material smoothly, avoiding abrupt changes in direction that can lead to tool breakage.
Challenges and Solutions in 3D Machining
3D machining involves complex contours and surfaces. Overcoming challenges in 3D machining requires advanced techniques and tools:
- 5-Axis Machining: Utilize 5-axis CNC machines to access difficult-to-reach areas and maintain consistent tool orientation.
- 3D Scanning and Modeling: Employ 3D scanning and modeling to digitize physical objects, creating accurate 3D representations that guide toolpath generation.
- High-Quality CAM Software: Invest in high-quality CAM software with 3D machining capabilities to generate toolpaths that follow intricate 3D shapes while minimizing tool movements.
Roughing and Finishing
Differentiating Between Roughing and Finishing Passes
Roughing and finishing passes serve distinct purposes in machining:
- Roughing: Roughing passes remove the bulk of material quickly, focusing on material removal rates rather than surface finish. Use larger tools and aggressive cutting parameters during roughing.
- Finishing: Finishing passes are designed to achieve the desired surface finish and dimensional accuracy. Use smaller, finer tools and precise cutting parameters during finishing.
Achieving Surface Finish and Dimensional Accuracy
To achieve the desired surface finish and dimensional accuracy:
- Stepover and Stepdown: Adjust stepover and stepdown values to control the tool’s engagement with the material, optimizing surface finish without compromising efficiency.
- Toolpath Compensation: Implement toolpath compensation techniques to account for tool deflection, ensuring dimensional accuracy and consistent part quality.
- Use of Finishing Tools: Employ specialized finishing tools, such as ball-nose end mills, for contouring and 3D surface finishing, achieving smoother surfaces.
Toolpath Simulation and Verification
Importance of Simulating Cutting Paths
Simulating cutting paths before actual machining is a crucial step to identify and rectify potential errors, collisions, or inaccuracies. Benefits of toolpath simulation include:
- Error Detection: Simulation helps detect errors in toolpath generation, preventing costly mistakes during actual machining.
- Collision Avoidance: Identifying collisions between the tool, workpiece, or fixtures allows for adjustments to prevent damage to the machine and workpiece.
- Optimization: Simulation provides an opportunity to optimize toolpaths further, improving machining efficiency and part quality.
Detecting and Correcting Errors Before Machining
When conducting toolpath simulation, it’s essential to analyze and address any detected errors or issues. This may involve:
- Collision Resolution: Modify toolpaths to avoid collisions by adjusting toolpath parameters or repositioning the workpiece or fixtures.
- Toolpath Adjustments: Fine-tune toolpaths to address issues like tool deflection, vibrations, or insufficient clearance between the tool and workpiece.
- Verification Protocols: Establish verification protocols to ensure that toolpaths meet design specifications, tolerances, and quality standards.
Designing effective cutting paths in CNC machining involves a multifaceted approach that considers material properties, geometric complexities, optimization techniques, 2D and 3D machining strategies, roughing and finishing passes, and the critical role of toolpath simulation and verification. By carefully addressing each of these aspects, manufacturers can optimize their machining processes, reduce costs, extend tool life, and consistently deliver high-quality components. Effective cutting path design is not only a science but also an art that empowers CNC machinists to turn raw materials into precise, intricate, and flawless finished products.
Advanced Cutting Path Strategies
In the ever-evolving world of CNC machining, advanced cutting path strategies have revolutionized precision, efficiency, and versatility. This section explores cutting-edge techniques, including High-Speed Machining (HSM), Adaptive Machining, Toolpath Smoothing and Optimization, Multi-Axis Machining, and the integration of Additive and Hybrid Manufacturing.
High-Speed Machining (HSM)
Principles of HSM and Its Benefits
High-Speed Machining (HSM) is a cutting-edge technique that has transformed the CNC machining landscape. Its principles and benefits include:
- Higher Cutting Speeds: HSM involves operating the CNC machine at significantly higher cutting speeds compared to conventional machining. This leads to reduced machining time, higher productivity, and cost savings.
- Lighter Cuts: HSM employs shallow cuts with high feed rates, reducing tool engagement and minimizing cutting forces. This approach enhances tool life and surface finish.
- Optimized Toolpaths: HSM employs optimized toolpaths that minimize sharp direction changes, reducing stress on the tool and workpiece.
- Superior Surface Finish: By maintaining high spindle speeds, HSM can achieve exceptional surface finishes, often eliminating the need for secondary finishing processes.
- Efficient Chip Evacuation: Increased feed rates in HSM promote efficient chip evacuation, reducing the risk of chip buildup and tool damage.
Challenges and Considerations in HSM
While HSM offers numerous advantages, it also presents unique challenges and considerations:
- Tool Wear: The higher cutting speeds in HSM can lead to accelerated tool wear. It’s crucial to select durable tool materials and coatings and monitor tool condition closely.
- Machine Rigidity: HSM requires CNC machines with high rigidity to withstand the increased cutting forces. Inadequate machine rigidity can lead to vibration, chatter, and poor surface finish.
- Cooling and Lubrication: Efficient cooling and lubrication systems are critical in HSM to manage the heat generated by higher cutting speeds and to prevent thermal damage to the workpiece and tool.
Adaptive Machining
Adaptive Toolpaths and Their Advantages
Adaptive Machining represents a dynamic approach to CNC machining that adjusts toolpaths in real-time based on the actual conditions encountered during machining. The advantages of adaptive machining include:
- Optimized Cutting Conditions: Adaptive toolpaths adapt to the changing conditions of the material and tool, optimizing cutting parameters such as depth of cut and feed rate for maximum efficiency and tool life.
- Reduced Tool Stress: By constantly adjusting to the workpiece’s material properties and geometry, adaptive machining minimizes tool stress, reducing the risk of tool breakage and wear.
- Improved Surface Finish: Adaptive machining can maintain consistent cutting conditions, resulting in superior surface finish, even when machining complex or variable-geometry parts.
- Reduced Cycle Times: The ability to adapt to the cutting environment leads to shorter machining cycle times, enhancing overall productivity.
Real-World Applications of Adaptive Machining
Adaptive machining finds applications in various industries, including aerospace, automotive, and medical device manufacturing:
- Aerospace: In aerospace, adaptive machining is used to efficiently machine complex airfoil shapes, reducing tool wear and improving the quality of critical components.
- Automotive: Adaptive machining is applied in the production of engine components, molds, and dies, where maintaining precise dimensions and surface finish is crucial.
- Medical Devices: The medical device industry benefits from adaptive machining when producing intricate, patient-specific implants and instruments with tight tolerances.
Toolpath Smoothing and Optimization
Algorithms for Optimizing Toolpaths
Toolpath smoothing and optimization involve applying advanced algorithms to toolpaths to achieve superior results in terms of machining quality and efficiency. Key aspects include:
- Curvature-Based Optimization: Algorithms analyze the curvature of toolpaths and smooth out sharp corners and sudden changes in direction, reducing the risk of chatter and vibration.
- Step Reduction: Toolpath optimization algorithms aim to minimize unnecessary steps and rapid tool movements, which can lead to inefficient machining and tool wear.
- Toolpath Compensation: These algorithms account for tool deflection, automatically adjusting toolpaths to achieve the desired dimensional accuracy.
Reducing Chatter, Vibrations, and Tool Deflection
Chatter, vibrations, and tool deflection are common issues in CNC machining. Toolpath smoothing and optimization techniques help mitigate these problems by:
- Minimizing Jerky Movements: Algorithms smooth toolpath trajectories, ensuring gradual transitions between movements and minimizing sudden direction changes.
- Stabilizing Cutting Forces: Toolpath optimization ensures even tool engagement, preventing overloading of certain areas and extending tool life.
- Balancing Tool Engagement: By optimizing cutting conditions, these techniques help stabilize cutting forces, reducing the risk of chatter and vibrations.
Multi-Axis Machining
Exploring Multi-Axis CNC Machines
Multi-axis machining takes CNC machining to a new level by incorporating machines with more than the traditional three axes (X, Y, and Z). These machines enable tool movement along additional rotational or tilting axes, offering unparalleled machining capabilities. Common types of multi-axis machines include 4-axis and 5-axis machines.
- 4-Axis Machining: In 4 axis machining, the workpiece can be rotated about an additional axis, usually referred to as the A-axis. This enables machining features on multiple sides of the workpiece without repositioning.
- 5-Axis CNC Machining: 5 axis machines add a rotary tilt table, often called the B-axis, to the capabilities of 4-axis machines. This allows for even more complex and intricate part geometries to be machined in a single setup.
Applications and Advantages of Multi-Axis Machining
Multi-axis machining is employed in various industries for its versatility and precision:
- Aerospace: Multi-axis machines are used to create complex aircraft components with intricate shapes and contours.
- Medical Devices: In medical device manufacturing, multi-axis machining is crucial for producing highly detailed and patient-specific implants and prosthetics.
- Automotive: Multi-axis machines are utilized to manufacture engine components, transmission parts, and complex molds and dies.
- Tool and Die Making: Multi-axis machining plays a vital role in the production of molds, dies, and tooling for various industries.
Additive and Hybrid Manufacturing
Integration of Additive Processes with CNC Machining
Additive Manufacturing (AM), often referred to as 3D printing, and CNC machining are often seen as complementary technologies. By integrating additive processes with CNC machining, a hybrid manufacturing approach emerges. Key considerations include:
- Design Freedom: Additive manufacturing allows for the creation of intricate and complex geometries that may be challenging to machine conventionally.
- Material Selection: Combining additive processes with CNC machining enables the use of a wider range of materials, including metals, plastics, and composites.
- Improved Efficiency: Hybrid manufacturing can optimize the production of complex parts, reducing material waste and machining time.
Advancements in Hybrid Manufacturing Technologies
Advancements in hybrid manufacturing have led to innovative solutions:
- In-Situ AM: Some hybrid machines incorporate additive manufacturing capabilities directly into CNC machining centers, allowing for in-situ 3D printing of features or toolpath optimization.
- Material Deposition and Removal: Hybrid machines are equipped with both additive and subtractive capabilities, enabling the deposition of material where needed before machining to achieve intricate geometries.
- Reduced Post-Processing: Hybrid manufacturing minimizes the need for extensive post-processing by creating parts with near-net shape geometry.
Advanced cutting path strategies in CNC machining, including High-Speed Machining (HSM), Adaptive Machining, Toolpath Smoothing and Optimization, Multi-Axis Machining, and the integration of Additive and Hybrid Manufacturing, have revolutionized the precision, efficiency, and capabilities of the industry. These cutting-edge techniques open new possibilities for machining complex parts, reducing cycle times, extending tool life, and pushing the boundaries of what is achievable in the world of manufacturing. As technology continues to advance, the integration of these strategies will play a pivotal role in shaping the future of CNC machining.
In Conclusion
In the world of CNC machining, the design and execution of cutting paths are fundamental to achieving precision, efficiency, and innovation. This comprehensive guide has explored the key principles and strategies associated with cutting paths, from the fundamentals to advanced techniques, highlighting their importance in modern manufacturing.
- Fundamentals of Cutting Paths explained the definition and significance of cutting paths in CNC machining, emphasizing their role in ensuring precision, efficiency, and cost-effectiveness. It also elucidated the relationship between cutting paths and toolpaths and introduced the coordinate systems used in CNC machining.
- Types of Cutting Paths delved into various cutting path types, such as contour cutting paths, pocketing, profiling, drilling, and hole-making paths. These distinctions are vital for selecting the appropriate path to match specific machining operations and part designs.
- Tool Selection and Geometry emphasized the significance of understanding tool geometries and their impact on machining performance. Proper tool selection considerations were also discussed, highlighting the importance of matching tools to material types and cutting conditions.
- Cutting Parameters elucidated the role of speed, feed rate, and depth of cut in shaping the cutting path and affecting machining efficiency. It emphasized the importance of balancing these parameters for optimal results.
- Toolpath Generation Software highlighted the role of CAM software in generating toolpaths, optimizing machining operations, and simulating the machining process. It introduced popular CAM software options and their features, showcasing their importance in CNC machining.
- Designing Effective Cutting Paths explored considerations for materials, geometric complexities, and optimization strategies. It provided insights into creating complex shapes, avoiding toolpath collisions, and achieving efficient, error-free machining operations. Additionally, it emphasized the importance of material properties in cutting path design and introduced best practices for machining various materials.
- Advanced Cutting Path Strategies delved into cutting-edge techniques such as High-Speed Machining (HSM), Adaptive Machining, Toolpath Smoothing and Optimization, Multi-Axis Machining, and the integration of Additive and Hybrid Manufacturing. These strategies open new horizons for precision, efficiency, and versatility in CNC machining, revolutionizing the industry.
As CNC machining continues to evolve, the knowledge and application of cutting path strategies will remain pivotal. The pursuit of efficiency, precision, and innovation will drive advancements in cutting path design, tooling, software, and machine capabilities. Whether it’s achieving intricate 2D profiles, tackling complex 3D geometries, or seamlessly integrating additive processes, CNC machining will continue to shape the future of manufacturing. With a solid understanding of cutting paths and a willingness to embrace advanced strategies, manufacturers can stay at the forefront of this dynamic industry, delivering high-quality, precise, and innovative products to meet the demands of tomorrow’s world.