The automotive industry is undergoing a paradigm shift, with a strong emphasis on sustainability, efficiency, and performance. One key aspect driving this change is the relentless pursuit of reducing component weight in vehicles. This article delves into the various strategies, materials, and technologies employed by automotive engineers to achieve weight reduction, ultimately contributing to enhanced fuel efficiency, improved safety, and a reduced environmental impact.
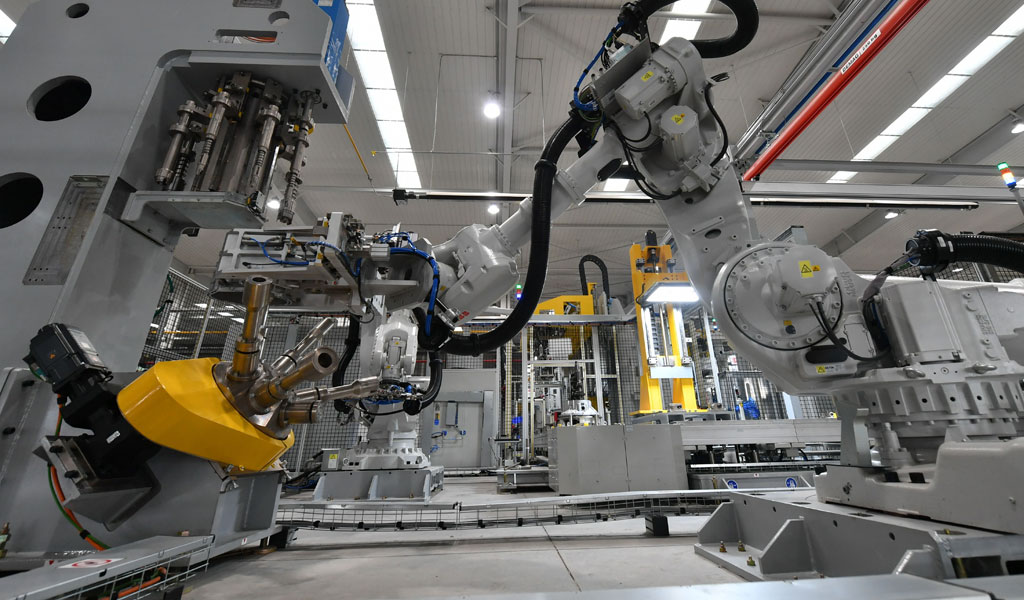
The Imperative for Weight Reduction in the Automotive Sector
The automotive industry, a dynamic and ever-evolving sector, plays a pivotal role in shaping the global economy and influencing technological advancements. In recent years, a compelling imperative has emerged within the industry: the relentless pursuit of reducing vehicle weight. This introductory section provides a concise overview of the current state of the automotive industry, highlighting the environmental impact of vehicle weight, regulatory pressures, and the prevailing trends that are reshaping the landscape of automotive design and manufacturing.
Current State of the Automotive Industry
As we navigate the 21st century, the automotive sector finds itself at a crossroads, facing challenges and opportunities that demand innovative solutions. The conventional paradigm of heavy, fuel-dependent vehicles is being reexamined, driven by factors such as rising concerns over climate change, energy efficiency, and the need for sustainable transportation solutions. Manufacturers and consumers alike are increasingly recognizing the importance of striking a balance between performance, safety, and environmental responsibility.
The Environmental Impact of Vehicle Weight
Vehicle weight is a critical factor influencing the environmental footprint of the automotive industry. Heavier vehicles typically consume more fuel, resulting in increased greenhouse gas emissions. Moreover, the extraction, production, and disposal of materials used in manufacturing contribute significantly to the industry’s overall environmental impact. Addressing the weight of vehicles is not merely a matter of operational efficiency but a crucial step towards mitigating the environmental consequences associated with the automotive lifecycle.
Regulatory Pressures and Industry Trends
Governments and regulatory bodies worldwide are enacting stringent standards aimed at reducing emissions and improving fuel efficiency. These regulations are propelling automakers to rethink traditional design and manufacturing approaches. The introduction of emission standards, fuel economy targets, and electric vehicle mandates are reshaping the industry’s trajectory. Additionally, consumers are increasingly inclined towards sustainable and eco-friendly choices, influencing automakers to incorporate lightweighting strategies into their product development.
Industry trends reflect a growing emphasis on electric and hybrid vehicles, autonomous driving technologies, and the integration of advanced materials. Lightweighting has emerged as a linchpin in achieving the goals set forth by these trends, as reducing vehicle weight directly contributes to increased energy efficiency, extended range for electric vehicles, and improved overall performance.
Milestones in Automotive Lightweighting
The journey towards lightweighting in the automotive industry can be traced back to pivotal milestones that shaped the trajectory of vehicle design. One such milestone was the introduction of mass-produced steel bodies in the early 20th century, replacing the heavier wooden structures of early automobiles. The 1920s witnessed the integration of aluminum in certain components, offering a notable reduction in weight without compromising structural integrity.
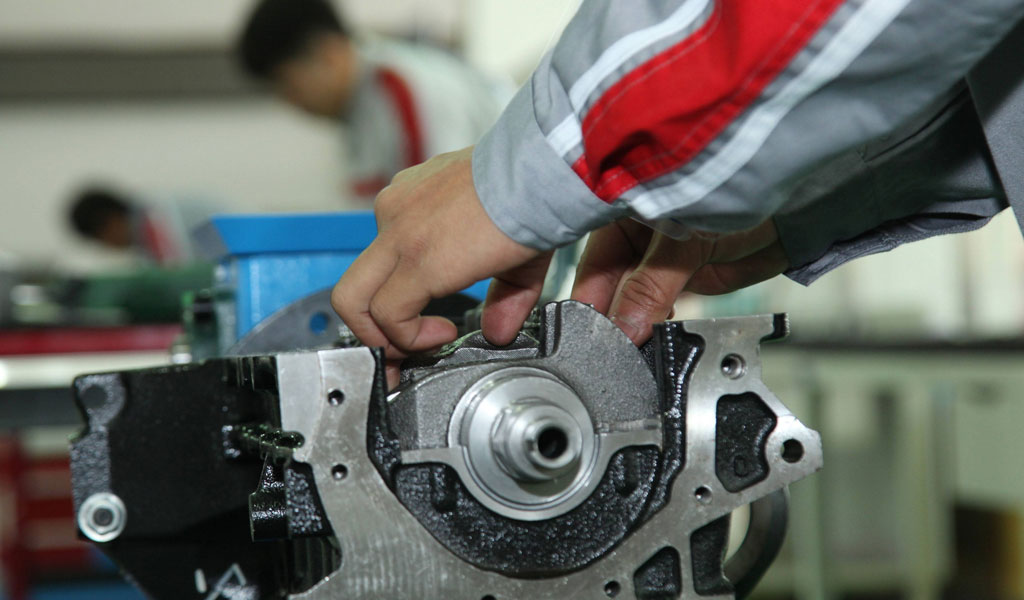
The post-World War II era brought about a surge in technological advancements and materials innovation. The utilization of fiberglass reinforced plastics (FRP) in sports car bodies, exemplified by the Chevrolet Corvette in the 1950s, marked a notable shift towards lightweighting for enhanced performance.
Early Materials and Design Philosophies
In the early stages of automotive design, materials such as cast iron and steel were predominant due to their strength and availability. However, these materials were inherently heavy, leading designers to explore alternatives. Aluminum, known for its lower density and corrosion resistance, became a material of interest, especially in high-performance and racing vehicles.
Design philosophies in the mid-20th century began emphasizing aerodynamics and streamlining to reduce drag and improve fuel efficiency. This era saw the integration of principles derived from aviation, as designers sought inspiration from aircraft structures to enhance the lightweight characteristics of automotive parts & components.
Technological Advancements Paving the Way for Modern Lightweighting
The latter half of the 20th century witnessed significant technological breakthroughs that laid the foundation for modern lightweighting strategies. The development of high-strength steel alloys in the 1970s marked a turning point, allowing manufacturers to maintain structural integrity while reducing overall weight. This advancement was particularly influential in the mass production of vehicles, balancing safety and efficiency.
The aerospace industry also played a crucial role in driving technological advancements. Carbon fiber composites, originally developed for aircraft, found their way into automotive applications in the 1980s and 1990s, revolutionizing the concept of lightweighting. Carbon fiber’s exceptional strength-to-weight ratio opened new possibilities for reducing the weight of critical components without compromising performance or safety.
Advancements in manufacturing technologies, such as stamping processes, laser cutting, and precision engineering, further facilitated the integration of lightweight materials into mainstream automotive production. These developments not only streamlined the manufacturing process but also made lightweighting more economically viable for a broader range of vehicles.
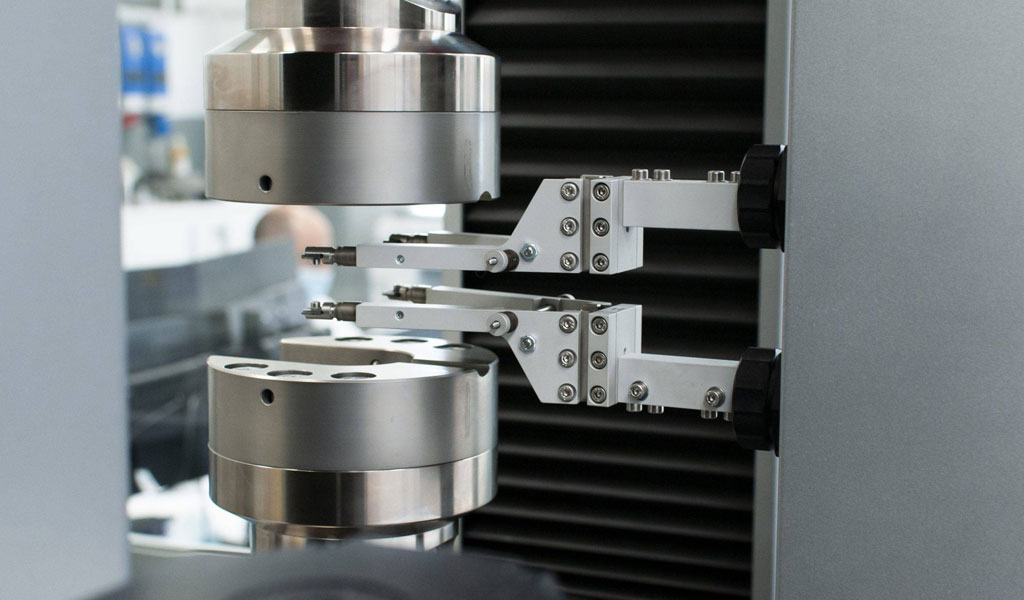
The Role of Advanced Materials in Weight Reduction
In the relentless pursuit of lightweighting in the automotive industry, the selection and utilization of advanced materials play a pivotal role.
This section examines several key materials that have revolutionized vehicle design, exploring their unique properties, applications, and the trade-offs involved in terms of cost, manufacturability, and performance.
High-Strength Steel Alloys
High-strength steel alloys have become stalwarts in the quest for weight reduction without compromising structural integrity. These alloys typically exhibit significantly higher strength-to-weight ratios than traditional steel, allowing for the creation of lighter yet durable components. Commonly used high-strength steels include advanced high-strength steel (AHSS) and ultra-high-strength steel (UHSS).
Applications: High-strength steel finds applications in critical structural components such as chassis, frame, and safety elements. The utilization of these alloys enhances crashworthiness and overall safety without adding excessive weight to the vehicle.
Trade-offs: While high-strength steel offers an excellent balance between strength and weight, there are trade-offs in terms of density and corrosion resistance compared to some alternative materials. Additionally, manufacturing high-strength steel components may require specialized processes, impacting overall production costs.
Aluminum and Its Alloys
Aluminum has long been a favorite in automotive lightweighting due to its low density and corrosion resistance. Aluminum alloys, which combine aluminum with other metals to enhance specific properties, offer a compelling solution for reducing overall vehicle weight.
Applications: Aluminum is commonly used in components such as body panels, chassis components, and engine parts. Its use significantly contributes to weight reduction, particularly in high-performance and electric vehicles where minimizing mass is critical for optimizing efficiency.
Trade-offs: Aluminum’s primary trade-off lies in its energy-intensive extraction and production processes. While it is lighter than steel, it may not provide the same structural strength. Additionally, aluminum’s higher cost and challenges in repairability compared to traditional materials are considerations in the material selection process.
Carbon Fiber Composites
Carbon fiber composites represent a paradigm shift in lightweighting, offering an exceptional strength-to-weight ratio and the ability to tailor material properties to specific applications. Comprising carbon fibers embedded in a polymer matrix, these composites have become synonymous with high-performance automotive applications.
Applications: Carbon fiber is widely used in components where weight reduction is critical, such as body panels, interior components, and structural elements. Its use in Formula 1 and high-end sports cars underscores its efficacy in achieving unparalleled lightweighting.
Trade-offs: The primary trade-offs with carbon fiber composites relate to cost and production complexity. Carbon fiber is an expensive material, and manufacturing processes involve intricate molding and curing steps. Repairability can also be challenging, impacting the overall cost of ownership.
Advanced Polymers and Plastics
The realm of advanced polymers and plastics encompasses a diverse range of materials, each with specific properties suitable for different applications. These materials, including reinforced polymers and engineering plastics, contribute to weight reduction while offering versatility in design.
Applications: Advanced polymers find applications in various components, such as interior trim, bumpers, and non-structural elements. Their use often provides a balance between weight reduction and cost-effectiveness.
Trade-offs: The trade-offs associated with advanced polymers and plastics involve considerations such as heat resistance, impact strength, and chemical resistance. While they offer advantages in terms of lightweighting, certain applications may require reinforcement or additional processing steps, impacting overall cost.
Trade-offs Between Materials: Cost, Manufacturability, and Performance
Selecting the appropriate material for a specific automotive component involves careful consideration of trade-offs between cost, manufacturability, and performance. High-strength steel alloys, for instance, offer a cost-effective solution with good performance but may pose challenges in manufacturing complexity. Aluminum provides a lightweight alternative but at a higher cost and potential trade-offs in repairability.
Carbon fiber composites offer unparalleled performance but come with higher production costs and complexities, while advanced polymers and plastics strike a balance between cost and weight reduction but may have limitations in certain performance aspects.
Manufacturers must weigh these trade-offs based on the intended application, performance requirements, and cost considerations. A holistic approach to material selection involves a thorough understanding of each material’s properties and how they align with the desired outcomes for the vehicle.
As we navigate the landscape of advanced materials in automotive lightweighting, the synergy between material science, engineering, and manufacturing becomes apparent. The subsequent sections will delve into advanced design strategies that leverage these materials to optimize weight reduction without compromising safety or performance.
Shaping the Future of Lightweight Automotive Components
The evolution of lightweighting in the automotive industry extends beyond the selection of advanced materials; it encompasses innovative design strategies aimed at maximizing the efficiency and performance of vehicles. This section explores key methodologies that are shaping the future of lightweight automotive components, including structural optimization, advanced simulation and modeling techniques, and the integration of materials with design for manufacturing (DFM) principles. Case studies will be presented to exemplify successful design strategies that have redefined the landscape of automotive engineering.
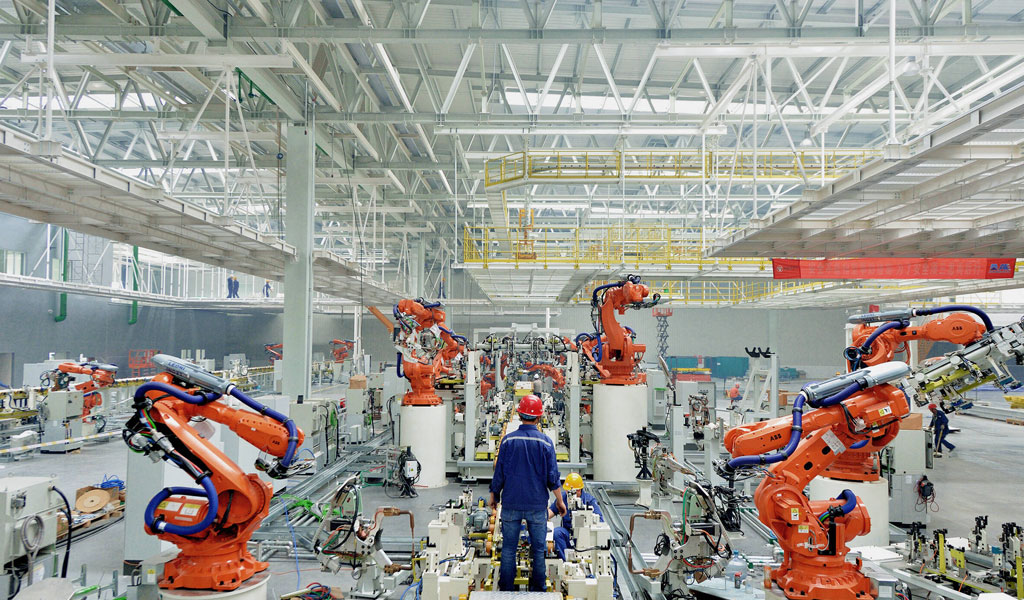
Structural Optimization and Topology Optimization
Structural optimization involves refining the shape and layout of components to achieve optimal strength and stiffness with minimal material usage. Topology optimization takes this concept further, exploring the most efficient distribution of material within a defined space. Both methodologies leverage advanced algorithms and computational tools to iteratively refine designs based on performance criteria.
Applications: These optimization techniques find applications in critical components such as chassis, suspension systems, and structural elements. By redistributing material in response to stress and load conditions, engineers can achieve weight reduction without compromising structural integrity.
Benefits: The benefits of structural and topology optimization include improved performance, enhanced efficiency, and reduced material waste. These methodologies empower designers to explore complex geometries that might not be intuitively apparent, pushing the boundaries of what is achievable in lightweighting.
Advanced Simulation and Modeling Techniques
Advanced simulation and modeling techniques enable engineers to assess the performance of components virtually before physical prototypes are produced. Finite Element Analysis (FEA), Computational Fluid Dynamics (CFD), and multi-body dynamics simulations provide invaluable insights into how lightweight components will behave under various conditions.
Applications: Simulation techniques are applied to diverse components, including body structures, suspension systems, and aerodynamic elements. These virtual tests help refine designs, ensuring they meet safety standards and performance criteria.
Benefits: The benefits of advanced simulation and modeling are multifaceted, ranging from cost savings associated with reduced prototype iterations to a deeper understanding of component behavior. Engineers can optimize designs iteratively, considering various factors such as stress distribution, heat dissipation, and aerodynamic efficiency.
Integration of Materials with Design for Manufacturing (DFM) Principles
Design for Manufacturing (DFM) is a holistic approach that considers the manufacturability of a component from the early stages of design. Integrating materials seamlessly with DFM principles involves understanding the capabilities and constraints of manufacturing processes and tailoring designs accordingly. This ensures that lightweight components can be produced efficiently and cost-effectively.
Applications: DFM principles are applied across the spectrum of automotive components, from complex structural elements to intricate interior features. The integration of materials with DFM ensures that lightweight designs align with practical manufacturing considerations.
Benefits: The integration of materials with DFM principles optimizes production processes, reduces lead times, and minimizes manufacturing costs. It facilitates a smoother transition from design concepts to real-world applications, fostering the practical implementation of lightweighting strategies.
Case Studies: Highlighting Successful Design Strategies
Case Study 1: BMW i3 Carbon Fiber Passenger Cell
- Overview: The BMW i3, an electric vehicle, features a passenger cell constructed predominantly from carbon fiber reinforced plastics (CFRP).
- Design Strategy: Structural optimization and topology optimization were employed to maximize the strength-to-weight ratio of the CFRP components, contributing to the vehicle’s overall lightweight design.
- Results: The BMW i3 exemplifies how advanced design strategies, coupled with innovative material choices, can redefine the possibilities for lightweight electric vehicles.
Case Study 2: Airbus A350 XWB Wing Design
- Overview: While not an automotive case study, the Airbus A350 XWB’s wing design illustrates the application of advanced simulation and modeling techniques.
- Design Strategy: Finite Element Analysis and aerodynamic simulations were extensively used to optimize the wing’s structural integrity and aerodynamic efficiency, contributing to significant weight reduction.
- Results: The A350 XWB showcases the potential of simulation-driven design in achieving lightweight components with enhanced performance in the aerospace sector, with lessons applicable to automotive design.
Case Study 3: Tesla Model S Aluminum Body
- Overview: The Tesla Model S features an aluminum body, contributing to the overall weight reduction of the vehicle.
- Design Strategy: Integration of materials with DFM principles played a crucial role in ensuring the manufacturability of the aluminum components, aligning design ambitions with practical production considerations.
- Results: The success of the Tesla Model S highlights the importance of a holistic approach that considers material choices alongside design and manufacturing principles for achieving lightweight yet robust automotive structures.
These case studies underscore the transformative impact of advanced design strategies in the automotive industry. As we delve further into the realm of lightweighting, the integration of these methodologies becomes increasingly essential, paving the way for a future where vehicles are not only lighter but also structurally efficient and technologically advanced.
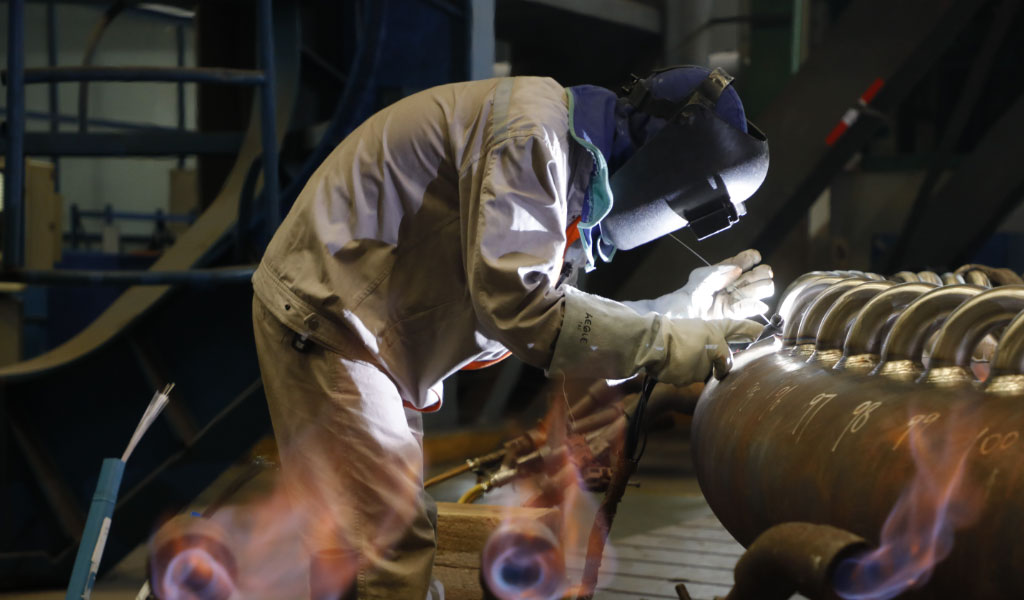
Revolutionizing Automotive Component Production
In the pursuit of lightweighting and innovative manufacturing processes, additive manufacturing technologies, commonly known as 3D printing, have emerged as transformative tools in the automotive industry. This section provides an in-depth overview of additive manufacturing technologies, explores their applications in automotive design, and discusses the benefits and challenges associated with adopting 3D printing for weight reduction.
Overview of Additive Manufacturing Technologies
Additive manufacturing refers to a group of technologies that build objects layer by layer, directly from digital models. These technologies have evolved significantly, offering diverse methods and materials for creating intricate and complex structures. Key additive manufacturing technologies include:
- Fused Deposition Modeling (FDM): This method involves extruding thermoplastic material layer by layer to create a three-dimensional object.
- Stereolithography (SLA): SLA uses a liquid resin cured by ultraviolet light to build up a model layer by layer.
- Selective Laser Sintering (SLS): SLS employs a laser to sinter powdered materials, such as plastics or metals, layer by layer.
- Direct Metal Laser Sintering (DMLS): Similar to SLS, DMLS uses a laser to sinter metal powders, enabling the production of metal components.
- PolyJet Printing: This technology jets layers of liquid photopolymer onto a build platform and cures each layer with ultraviolet light.
The Applications of 3D Printing in Automotive Design
The versatility of additive manufacturing technologies has led to their widespread adoption in various aspects of automotive design and production. The applications of 3D printing in the automotive industry are diverse and include:
- Prototyping: 3D printing allows for rapid prototyping of components, enabling engineers to test and refine designs quickly.
- Customization: Additive manufacturing facilitates the production of customized and complex components, such as interior features and personalized vehicle accessories.
- Tooling and Jigs: 3D printing is utilized in the creation of specialized tools, jigs, and fixtures used in the manufacturing process, enhancing efficiency and precision.
- Lightweight Structures: Additive manufacturing enables the creation of lightweight yet strong structures, reducing overall vehicle weight.
- Complex Geometries: The technology excels at producing intricate and complex geometries that may be challenging or impossible to achieve with traditional manufacturing methods.
Benefits of Adopting Additive Manufacturing for Weight Reduction
- Material Efficiency: Additive manufacturing allows for the precise placement of materials, minimizing waste and optimizing the use of resources.
- Complex Geometries: The ability to create intricate and complex geometries enables the design of lightweight structures that maintain strength and functionality.
- Customization: 3D printing facilitates the production of customized components, tailoring designs to specific applications and optimizing weight for individual needs.
- Rapid Prototyping: The speed at which prototypes can be produced and tested accelerates the design iteration process, leading to faster development cycles and, ultimately, weight-optimized components.
Challenges of Adopting Additive Manufacturing for Weight Reduction
- Material Limitations: While advancements continue, the range of materials suitable for additive manufacturing may be limited compared to traditional manufacturing methods.
- Production Speed: Despite rapid prototyping capabilities, the production speed of additive manufacturing may be a challenge for large-scale manufacturing processes.
- Post-Processing Requirements: Some 3D-printed components may require post-processing treatments to achieve the desired surface finish, adding complexity to the production workflow.
- Cost Considerations: Initial costs associated with acquiring and maintaining 3D printing equipment, as well as material costs, may be higher compared to traditional manufacturing methods.
Future Trends and Innovations in Additive Manufacturing
The continuous evolution of additive manufacturing technologies holds promising prospects for the automotive industry. Future trends may include the development of new materials with enhanced properties, increased production speed, and further integration of 3D printing into traditional manufacturing processes.
As we move forward, the automotive sector’s embrace of additive manufacturing technologies is poised to revolutionize component production, offering a pathway to more sustainable, efficient, and lightweight vehicles.
Smart Materials and Structural Innovations
As the automotive industry progresses towards more intelligent and efficient designs, the integration of smart materials and structural innovations is playing a pivotal role. This section explores key advancements in the field, including shape memory alloys, adaptive and responsive materials, morphing structures for aerodynamics and weight reduction, and the incorporation of sensors for real-time weight management.
Shape Memory Alloys
Shape memory alloys (SMAs) are materials that have the ability to return to a predetermined shape when subjected to a specific stimulus, typically a change in temperature or stress. In automotive applications, SMAs are employed to create components that can change shape or stiffness in response to external conditions.
Applications: SMAs find applications in various automotive components, including actuators for adaptive suspension systems, self-healing structures, and shape-shifting components such as active aerodynamic elements.
Benefits: The use of shape memory alloys contributes to improved vehicle performance, increased safety, and enhanced efficiency. SMAs can be utilized to create components that automatically adjust to changing conditions, optimizing the vehicle’s aerodynamics and overall functionality.
Adaptive and Responsive Materials
Adaptive and responsive materials refer to substances that can alter their properties or behavior in response to external stimuli, such as temperature, pressure, or electric fields. These materials enable the creation of components that can dynamically adapt to different driving conditions and environmental factors.
Applications: Adaptive and responsive materials are utilized in components like adaptive damping systems, variable stiffness structures, and intelligent surfaces that adjust their properties based on real-time data.
Benefits: The use of adaptive materials enhances vehicle performance, comfort, and safety. Components that can dynamically respond to changing conditions contribute to a more responsive and efficient driving experience.
Morphing Structures for Aerodynamics and Weight Reduction
Morphing structures involve the integration of components that can change shape to optimize aerodynamics, reduce drag, and enhance fuel efficiency. These structures are designed to adapt to varying driving conditions and speeds, providing a balance between stability and efficiency.
Applications: Morphing structures are applied in features like active aerodynamic surfaces, variable geometry spoilers, and adaptive wing configurations.
Benefits: The implementation of morphing structures contributes to improved aerodynamic performance, reduced fuel consumption, and increased overall vehicle efficiency. These structures allow for dynamic adjustments based on driving conditions, enhancing both performance and sustainability.
Incorporation of Sensors for Real-Time Weight Management
The integration of sensors for real-time weight management involves the use of sensors to monitor and manage the distribution of weight in a vehicle. This real-time data allows for dynamic adjustments to optimize handling, stability, and fuel efficiency.
Applications: Sensors are incorporated into various vehicle components, such as suspension systems, chassis, and load-bearing structures, to monitor weight distribution and make real-time adjustments.
Benefits: Real-time weight management enhances vehicle safety, stability, and overall performance. By dynamically adapting to changing weight conditions, the vehicle can optimize its handling characteristics and reduce unnecessary fuel consumption.
Future Trends in Smart Materials and Structural Innovations
The future of smart materials and structural innovations in the automotive industry holds exciting possibilities. Advancements in materials science, coupled with the integration of artificial intelligence and advanced sensor technologies, are likely to lead to even more sophisticated and intelligent automotive designs. The continuous exploration of smart materials and structural innovations is a testament to the industry’s commitment to creating vehicles that are not only lightweight but also adaptable, responsive, and technologically advanced.
Advanced Systems for Weight Reduction and Efficiency
In the pursuit of weight reduction and enhanced efficiency in automotive design, the integration of advanced electronic systems has become a key focus. This section explores how the integration of electronic components reduces mechanical complexity, the development of lightweight wiring harnesses and connectors, and the implementation of intelligent systems for weight distribution and balance.
Integration of Electronic Components to Reduce Mechanical Complexity
The integration of electronic components into vehicle systems has significantly contributed to reducing mechanical complexity. Traditional mechanical systems often involve intricate configurations of gears, levers, and linkages. The adoption of electronic control units (ECUs) and sensors replaces many of these mechanical components with electronic systems, streamlining designs and improving overall efficiency.
Applications: Electronic components are integrated into various vehicle systems, such as throttle control, braking, and steering. By replacing mechanical linkages with electronic systems, manufacturers can achieve more precise control and reduce the weight associated with traditional mechanical components.
Benefits: The integration of electronic components results in reduced mechanical complexity, increased reliability, and enhanced performance. It also allows for more sophisticated control strategies, contributing to improved fuel efficiency and safety.
Lightweight Wiring Harnesses and Connectors
Traditional wiring harnesses in vehicles are often heavy and complex due to the multitude of wires required for various electrical functions. The development of lightweight wiring harnesses and connectors involves utilizing advanced materials and design principles to reduce the overall weight and complexity of the electrical systems in vehicles.
Applications: Lightweight wiring harnesses and connectors are applied throughout the vehicle, including in the engine compartment, interior electronics, and lighting systems. Streamlining these components contributes to weight reduction without compromising functionality.
Benefits: The use of lightweight materials and innovative designs in wiring harnesses and connectors results in reduced vehicle weight, improved fuel efficiency, and simplified assembly processes. Additionally, advancements in materials enhance durability and resistance to environmental factors.
Intelligent Systems for Weight Distribution and Balance
Intelligent systems for weight distribution and balance involve the use of sensors, actuators, and control algorithms to dynamically adjust the distribution of weight within a vehicle. These systems aim to optimize handling, stability, and overall performance by actively managing weight distribution based on driving conditions.
Applications: Intelligent weight distribution systems are applied in various components, including adaptive suspension systems, active anti-roll bars, and dynamic stability control systems. These components work in tandem to ensure optimal weight distribution during acceleration, braking, and cornering.
Benefits: The implementation of intelligent weight distribution systems enhances vehicle dynamics, providing better handling, stability, and safety. By actively managing weight distribution, these systems contribute to improved overall performance and responsiveness.
Future Trends in Advanced Systems for Weight Reduction and Efficiency
The integration of advanced electronic systems, lightweight wiring components, and intelligent weight distribution technologies represents a dynamic field with ongoing innovations. Future trends are likely to include:
- Advanced Sensor Technologies: Continued advancements in sensor technologies will enable more precise monitoring of vehicle dynamics and weight distribution, allowing for even more sophisticated control strategies.
- Integration of Artificial Intelligence (AI): The use of AI algorithms in vehicle control systems will become more prevalent, enabling real-time decision-making for optimal weight distribution and efficiency.
- Electrification and Autonomy: As vehicles become more electrified and autonomous, the integration of electronic systems will play an even more central role in achieving weight reduction and efficiency gains.
- Materials Innovation: Ongoing research into lightweight materials for wiring and electronic components will contribute to further weight reduction without sacrificing functionality.
The ongoing evolution of advanced systems for weight reduction and efficiency reflects the automotive industry’s commitment to creating vehicles that are not only lighter but also smarter, more responsive, and technologically advanced.
Balancing Performance and Environmental Impact
Achieving a harmonious balance between performance and environmental impact is a critical imperative in the automotive industry. This section explores the methods and considerations involved in assessing the life cycle of lightweight materials, emphasizes the importance of recyclability and end-of-life considerations, and discusses the pivotal role of sustainable manufacturing processes in creating environmentally conscious vehicles.
Life Cycle Assessments of Lightweight Materials
Life cycle assessments (LCAs) are comprehensive evaluations that consider the environmental impact of a product or material throughout its entire life cycle, from raw material extraction to manufacturing, use, and eventual disposal. When applied to lightweight materials, LCAs help quantify the environmental footprint and overall sustainability of incorporating such materials in vehicles.
Applications: LCAs are conducted on various lightweight materials, including high-strength steel, aluminum, carbon fiber composites, and advanced polymers. Assessments compare factors such as energy consumption, greenhouse gas emissions, and resource depletion across the life cycle.
Benefits: The use of LCAs aids in informed decision-making, allowing manufacturers to select materials that minimize environmental impact without compromising performance. It provides a holistic view, guiding the industry towards sustainable choices in material selection.
Recyclability and End-of-Life Considerations
The recyclability of automotive materials is a crucial aspect of reducing the environmental impact of vehicles. Considering the end-of-life phase involves planning for the disposal or recycling of vehicles, ensuring that materials can be reused or reclaimed in an environmentally responsible manner.
Applications: Automotive manufacturers are increasingly focusing on designing vehicles with materials that are easily recyclable. This includes considerations for the separation of materials during the recycling process to maximize the recovery of valuable resources.
Benefits: Emphasizing recyclability and end-of-life considerations minimizes the environmental burden associated with automotive waste. Recyclable materials can be repurposed, reducing the demand for new raw materials and decreasing the overall ecological footprint of vehicle production.
The Role of Sustainable Manufacturing Processes
Sustainable manufacturing processes encompass a range of practices aimed at minimizing the environmental impact of production operations. In the context of automotive manufacturing, adopting sustainable practices involves reducing energy consumption, minimizing waste, and incorporating eco-friendly materials and technologies.
Applications: Sustainable manufacturing practices are implemented in various stages of automotive production, from the fabrication of components to final assembly. This includes the use of renewable energy sources, efficient waste management systems, and the incorporation of recycled materials.
Benefits: Sustainable manufacturing processes contribute to the reduction of greenhouse gas emissions, resource conservation, and overall environmental stewardship. They align with broader corporate social responsibility goals and address the growing consumer demand for eco-friendly products.
Future Trends in Balancing Performance and Environmental Impact
As the automotive industry continues to prioritize sustainability, several future trends are expected to emerge:
- Circular Economy Practices: The adoption of circular economy principles, where materials are reused and recycled in a closed-loop system, will become more prevalent in the automotive sector.
- Advanced Recycling Technologies: Continued advancements in recycling technologies, such as chemical recycling and innovative separation methods, will enhance the efficiency and effectiveness of material recovery from end-of-life vehicles.
- Regulatory Drivers: Stringent environmental regulations and evolving standards will compel manufacturers to invest in sustainable practices and materials, influencing the entire automotive supply chain.
- Collaboration and Industry Initiatives: Collaborative efforts among automotive manufacturers, suppliers, and regulatory bodies will drive industry-wide initiatives to promote sustainability, sharing best practices and innovations.
Achieving a balance between performance and environmental impact is not only a responsibility but also a strategic imperative for the automotive industry. The integration of life cycle assessments, recyclability considerations, and sustainable manufacturing practices is instrumental in shaping a future where vehicles are not only high-performing but also environmentally sustainable.
Conclusion: The Ongoing Journey Towards Sustainable, Lightweight Mobility
In the dynamic landscape of the automotive industry, the pursuit of sustainable, lightweight mobility stands as a guiding principle shaping the future of vehicle design and manufacturing. This journey involves a multifaceted approach encompassing materials innovation, advanced design strategies, electronic integration, and a commitment to environmental responsibility. As we conclude this exploration, let’s summarize the key takeaways and underscore the integral role of lightweighting in the future of automotive design.Key Takeaways:
- Evolution of Lightweighting: The historical evolution of lightweighting has witnessed significant milestones, from the introduction of high-strength steel to the integration of advanced materials such as carbon fiber composites. These advancements have reshaped the automotive landscape, offering a paradigm shift towards more sustainable and efficient vehicles.
- Advanced Materials: The integration of advanced materials, including high-strength steel alloys, aluminum, carbon fiber composites, and advanced polymers, plays a pivotal role in achieving weight reduction without compromising safety and performance. Each material brings unique characteristics and trade-offs, necessitating a holistic approach to material selection.
- Innovative Design Strategies: Structural optimization, advanced simulation techniques, and the integration of materials with design for manufacturing (DFM) principles represent innovative design strategies that contribute to the ongoing lightweighting revolution. These methodologies optimize vehicle performance, enhance safety, and accelerate the development of more sustainable automotive solutions.
- Additive Manufacturing: 3D printing technologies have revolutionized automotive component production, offering unparalleled flexibility in design and the potential for significant weight reduction. The integration of additive manufacturing aligns with the industry’s commitment to efficiency, customization, and reduced environmental impact.
- Smart Materials and Electronics: The incorporation of smart materials, such as shape memory alloys, and advanced electronic systems contribute to both weight reduction and improved vehicle performance. These technologies enable dynamic adjustments, adaptability to changing conditions, and enhanced efficiency.
- Sustainability at Every Stage: Achieving sustainable, lightweight mobility involves considerations at every stage of a vehicle’s life cycle. Life cycle assessments, recyclability, end-of-life considerations, and sustainable manufacturing processes collectively contribute to reducing the environmental impact of automotive production and usage.
The Integral Role of Lightweighting in the Future of Automotive Design
As we look ahead, lightweighting emerges as a cornerstone in the future of automotive design. The imperative to balance performance with environmental impact requires a strategic and holistic approach, with lightweighting serving as a catalyst for achieving these goals. The integration of advanced materials, smart technologies, and sustainable practices positions lightweighting at the forefront of innovations that redefine the automotive landscape.
From electric and hybrid vehicles to autonomous driving technologies, the weight of vehicles influences not only efficiency and range but also the overall environmental footprint. Lightweighting becomes not just a design strategy but a commitment to creating vehicles that are responsive, sustainable, and adaptable to the evolving demands of modern transportation.
Encouraging Continued Research and Innovation
The journey towards sustainable, lightweight mobility is ongoing, and its success hinges on continued research and innovation. The automotive industry must remain committed to exploring new materials, advancing design methodologies, and embracing cutting-edge technologies. Collaboration between manufacturers, researchers, and policymakers will play a pivotal role in fostering an environment where sustainability and efficiency are at the forefront of automotive advancements.
Encouraging a culture of innovation, open collaboration, and a shared commitment to environmental stewardship will propel the automotive industry towards a future where vehicles are not merely modes of transportation but symbols of progress, responsibility, and a sustainable tomorrow.
In conclusion, the ongoing journey towards sustainable, lightweight mobility signifies a transformative era in automotive history. It is a journey that holds the promise of not only redefining the vehicles we drive but reshaping the very foundations of how we approach transportation. As we navigate this path, let us remain vigilant in our pursuit of innovations that propel us towards a future where mobility is not just efficient and high-performing but also a responsible and sustainable part of our global ecosystem.