In recent years, the field of medical materials has witnessed groundbreaking advancements, with researchers and engineers constantly pushing the boundaries of innovation. One such material that has garnered significant attention is ultra-fine Nitinol. Nitinol, a shape memory alloy composed of nickel and titanium, has been widely used in various industries. However, its application in the medical field, particularly in the form of ultra-fine particles, opens up new possibilities for medical devices and treatments. This article delves into the unique properties of ultra-fine Nitinol and explores its diverse range of medical applications, from diagnostics to therapeutic interventions.
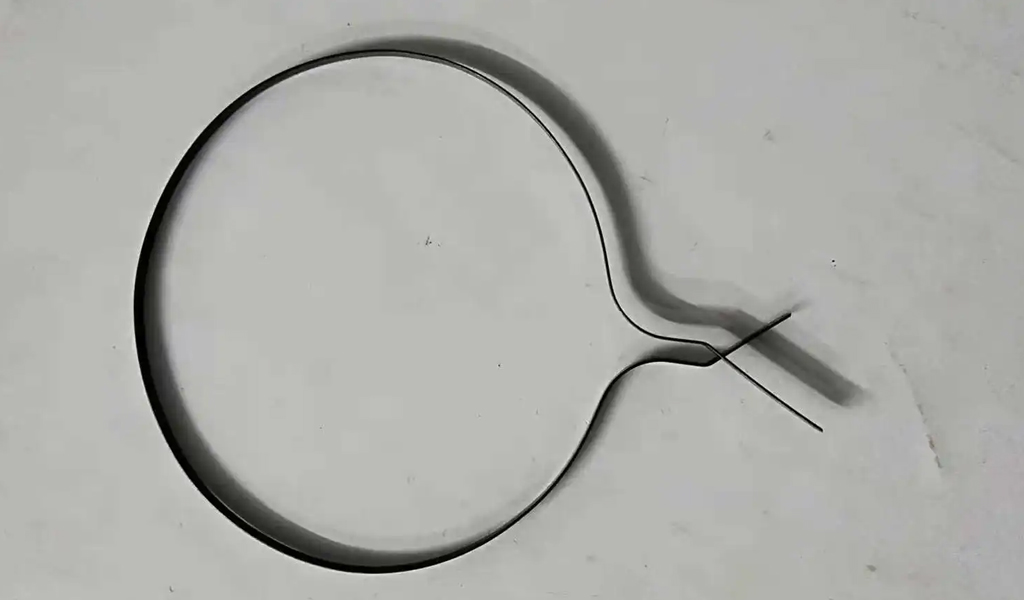
What Is Ultra-Fine Nitinol
Ultra-fine Nitinol refers to an advanced form of Nitinol, a shape memory alloy composed primarily of nickel and titanium.
Nitinol derives its name from a combination of these two elements and the Naval Ordnance Laboratory where it was initially discovered.
The alloy exhibits unique properties, making it highly valuable across various industries, particularly in the medical field.
The distinguishing feature of ultra-fine Nitinol lies in its particle size, which is reduced to the nanoscale. This refinement offers a range of advantages, especially in medical applications, where the nanosize particles enhance biocompatibility and enable novel functionalities. Understanding the key characteristics of ultra-fine Nitinol provides insights into its potential applications and contributions to medical technology.
Contact Be-Cu Today for Laser Cut Nitinol
Even though this material has become popular, it is not the easiest substrate to work with. Nitinol is a very reactive material. Because it responds to heat quickly, it has to be cut at relatively low temperatures in order to achieve the best results; however, if this material is allowed to cool too much, it could be formed, leading to warping, discoloration, and contamination. Therefore, you have to rely on a professional team that can prevent this from happening.
One way to do that is through laser cutting. That way, you end up with smooth lines despite complex cuts that will help you complete your project. At BE-CU.com, we use a carbon dioxide laser to help you get the best results. Our professionals have been specifically trained to work with this material, ensuring that you get the best results possible.
At Be-Cu , we always place your needs ahead of our down. Our professionals will work with you personally, answering any questions you may have. If you would like to learn more about our laser cutting nitinol services, it would be our pleasure to work with you. If you want quality, fast, and cost-effective results, contact us today.
The History Of Ultra-Fine Nitinol
The history of ultra-fine Nitinol is closely intertwined with the broader narrative of Nitinol’s discovery and development, which dates back to the 1960s. The unique properties of Nitinol, such as shape memory and superelasticity, have made it a sought-after material in various industries, with its medical applications being particularly noteworthy. The evolution of ultra-fine Nitinol represents a more recent chapter in the alloy’s history, marked by advancements in materials science and nanotechnology.
Discovery of Nitinol:
1960s: Nitinol’s story begins in the early 1960s when researchers at the Naval Ordnance Laboratory (now the Naval Surface Warfare Center) discovered an unusual alloy with exceptional properties. This alloy, composed of approximately equal parts of nickel and titanium, exhibited shape memory and superelasticity. The name “Nitinol” was derived from its components and the laboratory where it was first identified.
1970s: Throughout the 1970s, Nitinol found applications in various fields, including the aerospace industry and consumer products. Its ability to return to a predetermined shape after deformation and its resistance to fatigue made it a valuable material for engineering applications.
Medical Applications of Nitinol:
1980s-1990s: Nitinol’s medical applications began to gain prominence in the 1980s and 1990s. The alloy’s superelasticity and shape memory properties proved particularly beneficial in the development of medical devices, including vascular stents. The ability of Nitinol stents to be compressed for delivery and then expanded to their original shape in the body revolutionized minimally invasive procedures.
2000s: As Nitinol became widely adopted in the medical field, researchers and engineers began exploring ways to further enhance its properties for specific medical applications. This led to the development of ultra-fine Nitinol, where a focus on reducing particle size to the nanoscale opened up new possibilities for medical devices and treatments.
Emergence of Ultra-Fine Nitinol:
Advancements in Nanotechnology: The emergence of ultra-fine Nitinol can be attributed to advancements in nanotechnology. As researchers gained better control over materials at the nanoscale, they started exploring ways to manipulate Nitinol particles to achieve enhanced properties. The reduced size of these particles offered advantages in terms of biocompatibility, surface area, and interaction with biological tissues.
Improved Biocompatibility: The nanoscale dimensions of ultra-fine Nitinol resulted in improved biocompatibility, reducing the risk of adverse reactions when used in medical applications. The smaller size facilitated more efficient integration with biological tissues, opening new avenues for targeted drug delivery and implantable medical devices.
Current Developments and Research:
Ongoing Exploration: The history of ultra-fine Nitinol is a continuum of ongoing research and exploration. Researchers continue to investigate novel applications, such as targeted drug delivery, improved diagnostic imaging, and advanced therapeutic interventions. The potential of ultra-fine Nitinol in personalized medicine and the development of next-generation medical devices remains an active area of investigation.
Challenges and Future Prospects:
Biocompatibility Studies: The development of ultra-fine Nitinol brings challenges, including the need for thorough biocompatibility studies to ensure its safe integration into the human body. Long-term safety assessments, standardization of manufacturing processes, and regulatory approvals are crucial considerations for its widespread adoption in medical applications.
The history of ultra-fine Nitinol is a testament to the continuous evolution of materials science and the pursuit of innovative solutions in medicine. From its discovery at the Naval Ordnance Laboratory to the development of ultra-fine particles in recent years, Nitinol has made significant contributions to medical technology, and ongoing research promises even more exciting possibilities in the future.
The Type Of Ultra-Fine Nitinol
The classification of ultra-fine Nitinol involves categorizing the material based on various attributes, including its physical properties, particle size, and intended applications. Here is a classification framework for understanding and organizing ultra-fine Nitinol:
Particle Size:
Nanostructure: Ultra-fine Nitinol is characterized by its nanoscale particle size, typically ranging from a few nanometers to a few hundred nanometers. The specific size distribution can influence the material’s behavior and interactions at the molecular and cellular levels.
Subcategories: Depending on the specific application, ultra-fine Nitinol can be further classified into subcategories based on particle size, such as fine nanoparticles, ultrafine nanoparticles, and nanoclusters.
Composition and Alloy Characteristics:
Standard Nitinol Composition: Ultra-fine Nitinol maintains the fundamental composition of nickel and titanium in approximately equal proportions. The alloy’s composition remains a critical aspect of its classification, as variations may impact its physical and mechanical properties.
Alloy Modifications: Researchers may explore alloy modifications to tailor specific properties of ultra-fine Nitinol for particular applications. This can include alloying with other elements or adjusting the nickel-to-titanium ratio.
Surface Modifications and Functionalization:
Functionalized Ultra-Fine Nitinol: Depending on the desired application, ultra-fine Nitinol may undergo surface modifications or functionalization. This involves attaching specific molecules, ligands, or coatings to the surface of the nanoparticles to impart additional functionalities, such as improved biocompatibility, targeted drug delivery, or enhanced imaging capabilities.
Bioactive Coatings: Surface modifications may include bioactive coatings that promote specific biological responses, such as tissue integration, reduced inflammation, or enhanced cellular adhesion.
Magnetic Properties:
Magnetic Nanoparticles: Some classifications may be based on the magnetic properties of ultra-fine Nitinol. This is particularly relevant when the material is utilized in hyperthermia treatment or other therapeutic interventions that leverage its magnetic characteristics.
Magnetic Resonance Imaging (MRI) Enhancement: Ultra-fine Nitinol particles may be designed to enhance contrast in MRI by incorporating magnetic elements that interact with magnetic fields during imaging.
Biocompatibility and Toxicity:
Biocompatible Ultra-Fine Nitinol: Classification may consider the biocompatibility of ultra-fine Nitinol, with variations in surface properties and coatings to enhance compatibility with biological tissues. Evaluating potential toxicity is crucial for medical applications, influencing the material’s classification and regulatory considerations.
Bioresorbable Nitinol: In some cases, ultra-fine Nitinol may be designed to be bioresorbable, allowing it to gradually degrade over time within the body. This feature is particularly relevant for certain implantable medical devices.
The classification of ultra-fine Nitinol involves considering particle size, composition, surface modifications, intended applications, magnetic properties, and biocompatibility. This systematic categorization helps researchers, engineers, and healthcare professionals better understand and utilize the unique characteristics of ultra-fine Nitinol for diverse medical applications.
The Applications for Nitinol
Nitinol, a unique shape memory alloy composed of nickel and titanium, has found a wide range of applications across various industries due to its distinctive properties. The alloy’s ability to return to a predetermined shape after deformation and exhibit superelasticity makes it particularly valuable. Here are some notable applications for Nitinol:
Medical Devices
- Vascular Stents: Nitinol is extensively used in the production of vascular stents. These small, flexible mesh-like tubes are inserted into narrowed or blocked blood vessels to maintain their patency. Nitinol’s superelasticity allows stents(By Stent Laser Cutting) to be compressed for delivery through catheters and then expanded to their original shape upon deployment.
- Guidewires and Catheters: Nitinol’s flexibility and superelastic properties make it an ideal material for guidewires and catheters used in various medical procedures, especially those involving intricate vascular pathways. The alloy’s ability to navigate through complex anatomical structures improves the safety and effectiveness of procedures.
- Orthopedic Implants: Nitinol’s shape memory and biocompatibility make it suitable for orthopedic implants. It can be used in bone plates, screws, and other devices for orthopedic surgeries, providing stability and minimizing the risk of implant-related complications.
- Dental Instruments: Nitinol’s flexibility and shape memory are advantageous in the manufacturing of dental instruments, such as archwires used in orthodontics. These archwires can apply controlled forces to move teeth into desired positions.
- Neurovascular Devices: Nitinol is employed in the development of neurovascular devices, including aneurysm treatment devices and flow diverters. Its properties enable the creation of devices that can adapt to the unique anatomical features of the neurovascular system.
Actuators and Robotics
- Actuators: Nitinol’s ability to undergo shape changes in response to temperature variations makes it suitable for actuators in various applications. These actuators are utilized in robotics, aerospace, and other fields where precise and controlled movements are required.
- Soft Robotics: Nitinol is used in soft robotics, a field that involves the design of robots with flexible and deformable structures. The alloy’s shape memory allows for the creation of soft robotic components that can change shape or perform specific tasks based on environmental conditions.
Eyeglass Frames
Flexible Frames: Nitinol’s superelasticity is utilized in the manufacturing of eyeglass frames. Frames made from Nitinol can withstand deformation and return to their original shape, offering increased durability and flexibility compared to traditional eyeglass materials.
Consumer Electronics
- Mobile Phone Antennas: Nitinol is employed in the creation of retractable antennas for mobile phones. The shape memory property allows the antenna to retract and extend as needed without causing damage or compromising signal quality.
- Earphones and Headphones: Nitinol wires are used in earphones and headphones due to their flexibility and durability. The alloy’s ability to withstand repeated bending and stretching makes it suitable for these applications.
Automotive Industry
Automotive Engine Components: Nitinol’s thermal properties make it suitable for certain automotive applications, such as components in engines that experience temperature variations. Nitinol’s ability to withstand repeated thermal cycles without degradation is advantageous in these contexts.
Aerospace Applications
Aerospace Actuators: Nitinol’s lightweight and shape memory properties are beneficial in aerospace applications, particularly in the development of actuators for aircraft components. The alloy’s ability to operate reliably in a range of temperatures is advantageous in this sector.
Textile Industry
Smart Fabrics: Nitinol fibers can be woven into textiles to create smart fabrics. These fabrics can change their structure or properties in response to temperature changes, providing applications in areas such as sportswear and adaptive clothing.
Educational Demonstrations
Science Demonstrations: Nitinol is often used in educational settings to demonstrate principles of shape memory alloys and superelasticity. Classroom demonstrations showcase the alloy’s ability to return to a memorized shape after deformation, providing a hands-on understanding of its unique properties.
Nitinol’s versatility and unique properties continue to inspire innovations in various fields, contributing to advancements in technology, healthcare, and beyond. Ongoing research and development efforts are likely to uncover new applications for this remarkable alloy.
Characteristics that Affect the Performance of Nitinol
Several key characteristics of Nitinol significantly influence its performance in various applications. Understanding these characteristics is crucial for optimizing the design and functionality of devices or systems that incorporate Nitinol. The key characteristics include:
Shape Memory Effect (SME)
- Definition: The shape memory effect is one of Nitinol’s most distinctive features. It allows the alloy to “remember” its original shape and return to that shape after being deformed when exposed to a specific temperature (martensitic phase) and then heated back to a higher temperature (austenitic phase).
- Influence on Performance: The efficiency and repeatability of the shape memory effect are critical for applications such as stents, guidewires, and actuators. The transition temperatures, known as the martensitic and austenitic finish temperatures, must be precisely tailored for the intended application.
Superelasticity
- Definition: Nitinol exhibits superelastic behavior, allowing it to undergo significant deformation and recover its original shape when the external force is removed. This property is due to the stress-induced phase transformation between martensite and austenite.
- Influence on Performance: Superelasticity is crucial in applications like stents, where the device must be compressed for delivery and then expanded to its original shape within the body. The stress-strain relationship and hysteresis during loading and unloading cycles are vital considerations for optimizing superelastic behavior.
Transition Temperatures
- Martensitic Start/Finish Temperatures: These temperatures define the beginning and completion of the phase transition from austenite to martensite during cooling.
- Austenitic Start/Finish Temperatures: These temperatures represent the initiation and conclusion of the phase transformation from martensite to austenite during heating.
- Influence on Performance: Precise control of these transition temperatures is essential for ensuring that Nitinol devices exhibit the desired shape memory and superelastic behaviors at specific operational temperatures, aligning with the physiological conditions of the application.
Hysteresis
- Definition: Hysteresis in Nitinol refers to the difference in temperature between the start and finish of the phase transition during heating and cooling cycles.
- Influence on Performance: Lower hysteresis is generally desirable for applications requiring precise control over the shape memory effect. It minimizes the temperature range needed for the material to return to its original shape, improving the efficiency and responsiveness of Nitinol devices.
Training and Annealing
- Training: Nitinol can be “trained” to memorize a specific shape through repeated deformation and heating cycles.
- Annealing: Heat treatment processes, such as annealing, can alter Nitinol’s microstructure and properties.
- Influence on Performance: The effectiveness of training and annealing processes influences the stability and repeatability of Nitinol’s shape memory and superelastic behaviors. Proper processing is crucial for achieving desired performance characteristics.
Fatigue Resistance
- Definition: Fatigue resistance refers to Nitinol’s ability to withstand repeated loading and unloading cycles without experiencing structural damage.
- Influence on Performance: Nitinol devices, especially those used in biomedical applications like stents, must exhibit high fatigue resistance to withstand the dynamic conditions within the body over an extended period.
Corrosion Resistance
- Definition: Nitinol’s resistance to corrosion is vital for applications in physiological environments, where exposure to bodily fluids may occur.
- Influence on Performance: Corrosion resistance ensures the long-term durability and biocompatibility of Nitinol devices. Surface treatments or coatings may be applied to enhance corrosion resistance.
Understanding and optimizing these characteristics are essential for maximizing the performance and reliability of Nitinol in specific applications, ranging from medical devices to actuators and beyond. Successful integration requires careful consideration of the intended use and environmental conditions.
Mechanical Properties – Tensile Strength, Elongation, And Surface Condition
Mechanical Property | Typical Value | Importance | Testing Method |
---|---|---|---|
Tensile Strength | High, around 500-900 MPa | Crucial for structural integrity and load-bearing applications | Tensile testing |
Elongation at Break | Typically 10-20% or higher | Significant for applications requiring repeated deformation, such as medical devices | Tensile testing |
Superelasticity | Demonstrates significant elastic deformation and recovery | Key for adaptive devices and applications requiring shape memory | Tensile testing, stress-strain curve analysis |
Surface Finish | Smooth surface required for specific applications | Influences biocompatibility, ease of deployment, and interaction with tissues | Visual inspection, surface roughness measurements |
Surface Treatments | Varied coatings or treatments for specific functionalities | Enhances corrosion resistance, biocompatibility, or imparts specific characteristics | Coating analysis, surface characterization techniques |
In Conclusion
The exploration of ultra-fine Nitinol represents a significant leap forward in the realm of materials science, particularly in its applications within the medical field. The unique properties of Nitinol, combined with its nanoscale dimensions, open doors to innovative solutions that can revolutionize diagnostics, therapeutics, and medical device technologies. As we reflect on the journey through the history, characteristics, classifications, and mechanical properties of ultra-fine Nitinol, several key points emerge:
Evolution from Discovery to Innovation
From its discovery at the Naval Ordnance Laboratory to the development of ultra-fine particles, Nitinol has evolved from a novel material with shape memory and superelasticity to a cutting-edge solution with nanoscale dimensions. This evolution highlights the dynamic nature of materials science and the continuous pursuit of innovation.
Medical Breakthroughs and Applications
The medical applications of ultra-fine Nitinol are particularly noteworthy, with the potential to redefine diagnostics and therapeutics. The material’s enhanced biocompatibility, surface characteristics, and unique magnetic properties offer unprecedented opportunities in personalized medicine, targeted drug delivery, and advanced imaging techniques.
Challenges and Considerations
The journey through the exploration of ultra-fine Nitinol also underscores the importance of addressing challenges. Biocompatibility studies, safety assessments, and adherence to ethical and regulatory standards are crucial considerations in the development and deployment of this advanced material in medical applications.
Versatility and Collaboration
Ultra-fine Nitinol’s versatility stems from its nanoscale dimensions, offering a wide range of possibilities across multiple disciplines. Collaboration between materials scientists, engineers, healthcare professionals, and regulatory bodies is paramount to harnessing its full potential.
Precision Manufacturing and Quality Control
The successful integration of ultra-fine Nitinol into medical applications requires precision manufacturing techniques and rigorous quality control. Attention to detail in the fabrication process ensures consistency and reliability, critical elements for the material’s success in real-world applications.
Continued Research and Future Prospects
The exploration of ultra-fine Nitinol is an ongoing journey marked by continuous research and development. The potential applications in diagnostics, therapeutics, and medical devices are vast, and future innovations are likely to emerge as our understanding of this material deepens.
In the grand tapestry of materials science, ultra-fine Nitinol stands as a testament to human ingenuity and the relentless pursuit of advancements that can benefit society. As we move forward, the collaboration of diverse disciplines, ethical considerations, and a commitment to quality will be essential in unlocking the full potential of ultra-fine Nitinol, ushering in a new era of medical technology and healthcare solutions.